Visualization of the Skeletal Muscle Stem Cell Niche in Fiber Bundles
Svenja C. Schüler, Svenja C. Schüler, Simon Dumontier, Simon Dumontier, Jonathan Rigaux, Jonathan Rigaux, C. Florian Bentzinger, C. Florian Bentzinger
Abstract
Skeletal muscle stem cells (MuSCs) reside in a complex niche composed of the muscle fiber plasma membrane and the laminin-rich basal lamina surrounded by the microvasculature, as well as different supportive cell types such as fibro-adipogenic progenitors residing in the interstitial extracellular matrix. Within the first few hours after tissue damage, MuSCs undergo cytoskeletal rearrangements and transcriptional changes that prime the cells for activation. Due to their time-consuming nature, enzymatic methods for liberation of single muscle fibers with fully quiescent MuSCs are challenging. Moreover, during enzymatic digestion, important niche components including the microvasculature and the collagenous interstitial matrix are destroyed. Here, we provide a method for the visualization of MuSCs on muscle fibers in their intact niche. Our method relies on mechanical teasing of fiber bundles from fixed skeletal muscles. We demonstrate that teased muscle fiber bundles allow the investigator to capture a representative snapshot of the MuSC niche in skeletal muscle, and outline how stem cell morphology and different microenvironmental components can be visualized. © 2021 The Authors. Current Protocols published by Wiley Periodicals LLC.
Basic Protocol 1 : Isolation of fiber bundles
Basic Protocol 2 : Immunofluorescence staining of MuSCs on fiber bundles
Support Protocol : Preparation of Sylgard dishes
INTRODUCTION
In adult skeletal muscle, quiescent MuSCs with extended cytoskeletal protrusions reside between the basal lamina and the muscle fiber plasma membrane. The MuSC niche is in close proximity to microvessels and supportive cell types such as fibro-adipogenic progenitors (FAPs) in the interstitial space (Joe et al., 2010). Upon injury, MuSCs undergo a cascade of intrinsically and extrinsically regulated molecular changes. Once activated, MuSCs retract cytoskeletal protrusions and begin to proliferate and eventually differentiate to form new muscle fibers. MuSC activation is paralleled by transcriptional cascades allowing for commitment to differentiation and down-regulation of genes maintaining quiescence (Bentzinger, Wang, & Rudnicki, 2012). Within the first hours of activation, the immediate early genes c-fos, c-myc, and c-jun begin to be expressed in MuSCs, and the majority of cells subsequently up-regulate the commitment factors Myf5 and MyoD, priming them for proliferation and differentiation (Machado et al., 2017; Schmidt, Schüler, Hüttner, von Eyss, & von Maltzahn, 2019; van Velthoven, de Morree, Egner, Brett, & Rando, 2017). MuSC differentiation is accompanied by increased expression of myogenin and Mrf4, while commitment factors are down-regulated. During regeneration, a sub-population of MuSCs that are lineage-negative for Myf5 self-renew to maintain the stem cell pool (Kuang, Kuroda, Le Grand, & Rudnicki, 2007). Once myogenesis is completed, MuSC quiescence is re-established following exposure to Notch ligands expressed by the microvasculature and other microenvironmental signals (Verma et al., 2018).
Apart from intrinsic mechanisms, MuSC fate decisions are controlled by stimuli originating from their niche microenvironment. These extracellular signals consist of paracrine factors, extracellular matrix (ECM), or cell-cell contacts, or are of a biomechanical nature. For instance, molecules secreted by macrophages, eosinophils, neutrophils, FAPs, and fibroblasts, among others, have been shown to regulate MuSC function (Bentzinger, Wang, Dumont, & Rudnicki, 2013). FAPs secrete Wisp1, a matricellular protein that stimulates MuSC commitment, as well as the ECM component fibronectin, which controls MuSC self-renewal in conjunction with Wnt7a released by immature muscle fibers in the regenerative niche (Bentzinger et al., 2013; Le Grand, Jones, Seale, Scime, & Rudnicki, 2009; Lukjanenko et al., 2019). Additionally, changes in the stiffness of the extracellular matrix regulated by structural ECM proteins such as Collagen VI have been shown to be critical for the maintenance of the adult MuSC pool (Urciuolo et al., 2013).
Enzymatic treatment of skeletal muscles using collagenase allows for the digestion of the interstitial collagen-rich ECM, liberating single fibers containing MuSCs under the basal lamina that can be analyzed directly or that can be cultured to monitor stem cell dynamics after activation (Bischoff, 1986; Pasut, Jones, & Rudnicki, 2013). However, the interstitial space contains numerous cell types known to regulate MuSC function, and destruction of the collagenous matrix also releases the microvasculature that is tightly associated with muscle fibers. Thus, enzymatically isolated single muscle fibers (EIFs) contain only niche components present under the basal lamina (Fig. 1A). Moreover, the time-consuming nature of protocols for enzymatic liberation support the notion that MuSCs on single fibers are not bona fide quiescent, but have already initiated activation.
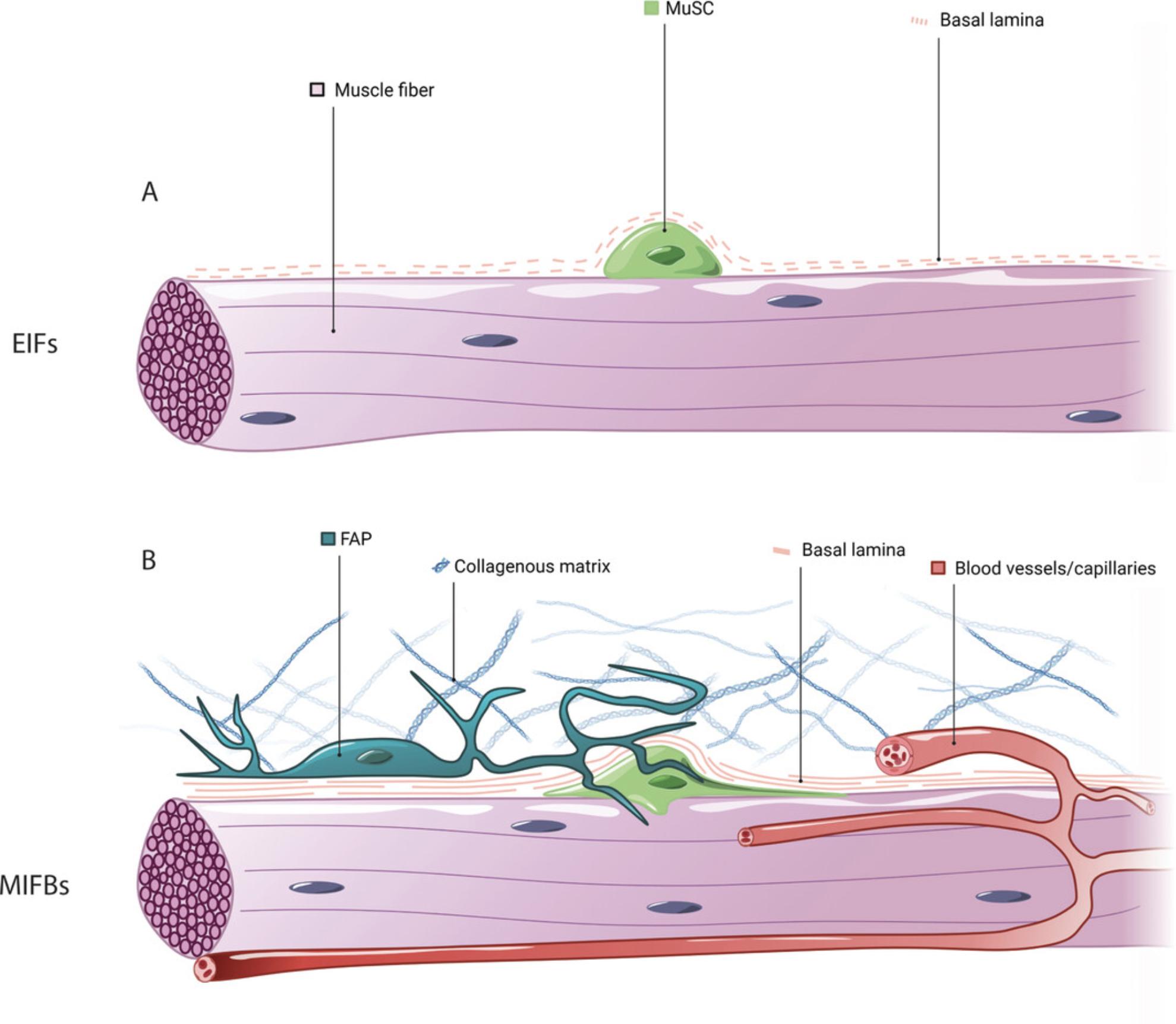
Complementing the enzymatic single-fiber method, mechanically isolated muscle fiber bundles (MIFBs) obtained from pre-fixed skeletal muscle allow for the investigation of MuSCs unaffected by ex vivo manipulation, and can be used to visualize the intact stem cell niche. Here, we outline the muscle fiber bundle method based on mouse extensor digitorum longus (EDL) muscles. After ex vivo fixation, muscle fiber bundles are teased by mechanical manipulation. In contrast to EIFs, MIFBs can be used to reveal niche components including the undamaged basal lamina, supportive interstitial cell types, and the morphology of quiescent MuSCs with cytoskeletal protrusions (Fig. 1B). We describe how to use antibodies for laminin, Pax7, and M-cadherin for the characterization of the basal lamina and MuSCs in fiber bundle preparations (Pouliot, Gravel, & Holland, 1994; Seale et al., 2000). In addition, based on antibody staining for CD31 and PDGFRα, we outline how the microvasculature and FAPs in the quiescent MuSC niche can be revealed in MIFBs (Joe et al., 2010; van Mourik, Leeksma, Reinders, de Groot, & Zandbergen-Spaargaren, 1985).
Basic Protocol 1—Isolation of fiber bundles—describes the isolation and fixation of the EDL muscle with subsequent separation into fiber bundles. Basic Protocol 2—Immunofluorescence staining of MuSCs on fiber bundles—describes immunofluorescence staining of MuSCs located on fiber bundles with antibodies to laminin, Pax7, and M-cadherin, as well as visualization of the microvasculature and FAPs using antibodies to CD31 and PDGFRα. The Support Protocol—Preparation of Sylgard dishes—describes the preparation of transparent Sylgard polymer dishes that are used for tissue manipulation during muscle fiber bundle isolation.
Basic Protocol 1: ISOLATION OF FIBER BUNDLES
This protocol describes the preparation of MIFBs from paraformaldehyde (PFA)−fixed EDL muscles for immunofluorescence analysis. Throughout the protocol, EDL muscles should be handled only by their tendons to prevent damage and contraction of fibers. After isolation, MIFBs can either immediately be used for immunofluorescence staining or can be stored in PBS at 4°C.
Materials
-
Mouse
-
70% ethanol
-
Fixation buffer (see recipe)
-
Phosphate-buffered saline (PBS), pH 7.4 (Bio Basic, cat. no. PD8117)
-
Iris scissors (Fine Science Tools Inc., cat. no. 14060-09)
-
Curved Dumont tweezers (Fine Science Tools Inc., cat. no. 11251-35)
-
Vannas scissors (Fine Science Tools Inc., cat. no. 15003-08)
-
Sylgard dish (see Support Protocol)
-
30-G needles (BD, cat. no. 305106)
-
Syringes (BD, cat. no. 309659)
-
27-G needles (BD, cat. no. 309623)
-
Binocular microscope
-
1.7-ml horse serum−coated reaction tubes (see recipe)
-
Additional reagents and equipment for euthanasia of rodents (see Current Protocols article: Donovan & Brown, 2006)
1.Euthanize the mouse according to animal welfare regulations (see Current Protocols article: Donovan & Brown, 2006).
2.If not done before, perform cervical dislocation (also described in Donovan & Brown, 2006) to rupture the spinal cord and neck vessels. This will lower pressure on the microvasculature and prevent bleeding into the muscle.
3.Spray the whole animal with 70% ethanol for sterile working conditions (Fig. 2A).
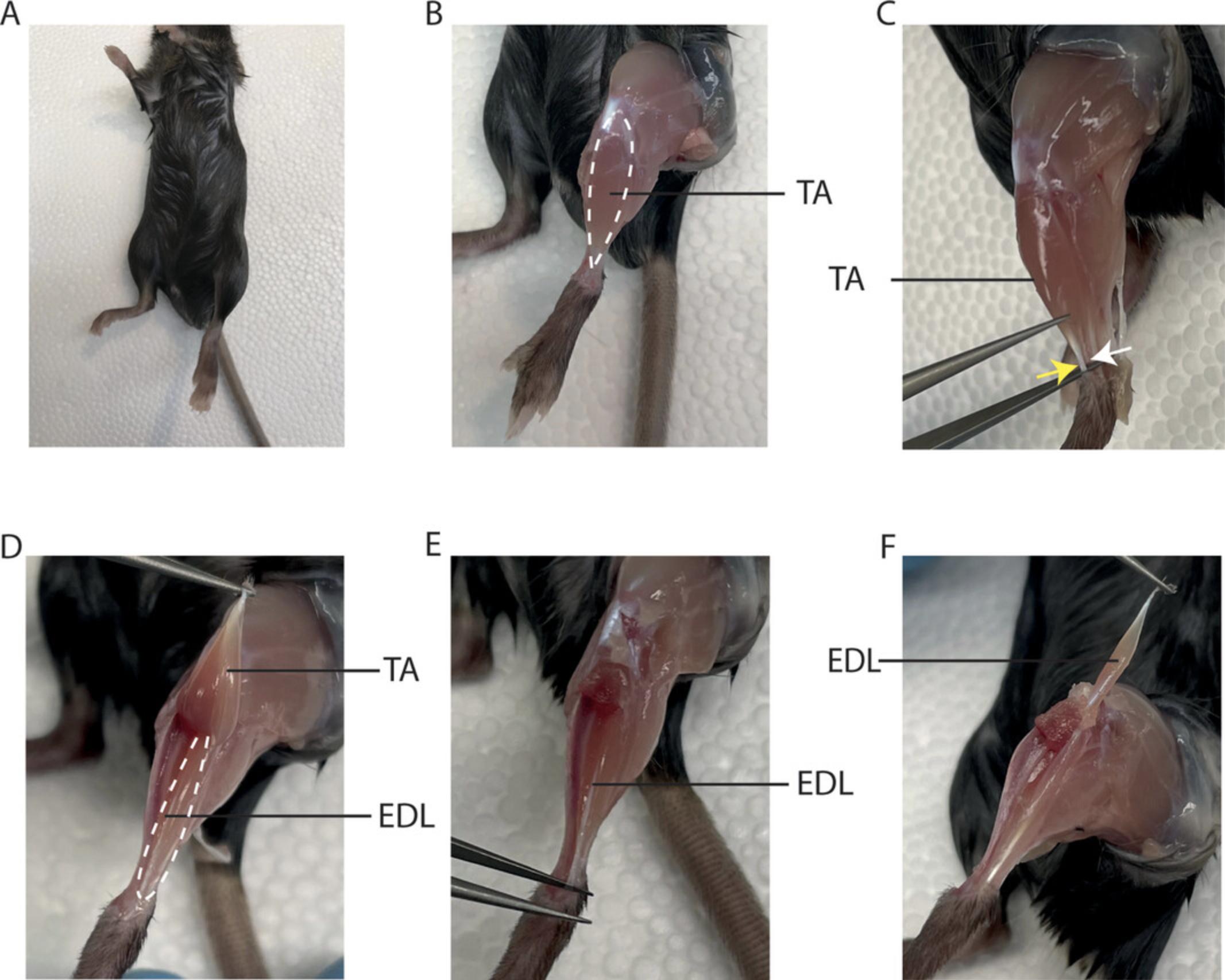
4.Cut the skin around the foot using iris scissors and pull it up to the hip using curved Dumont tweezers.
5.Remove the fascia by sliding curved Dumont tweezers from the foot towards the knee between the tibialis anterior (TA) muscle and the tibia (Fig. 2B).
6.Using curved Dumont tweezers, carefully grab the TA muscle tendon (yellow arrow) above the distal EDL tendon (white arrow). Be careful not to damage the EDL (Fig. 2C).
7.Cut the tendon of the TA muscle with Vannas scissors.
8.Using curved Dumont tweezers, pull the TA muscle to the knee and cut it off at the bone (Fig. 2D).
9.Using Vannas scissors, remove the tissue surrounding the knee to reveal the proximal EDL tendon.
10.Liberate the distal EDL tendon with curved Dumont tweezers and cut it with Vannas scissors (Fig. 2E).
11.Using curved Dumont tweezers, slowly pull the EDL muscle towards the knee. Cut the proximal tendon connecting the EDL and the knee with Vannas scissors (Fig. 2F).
12.Place the EDL in a dish filled with hardened transparent Sylgard. Using 30-G needles, pin the tendons under light tension to the dish (Fig. 3A).
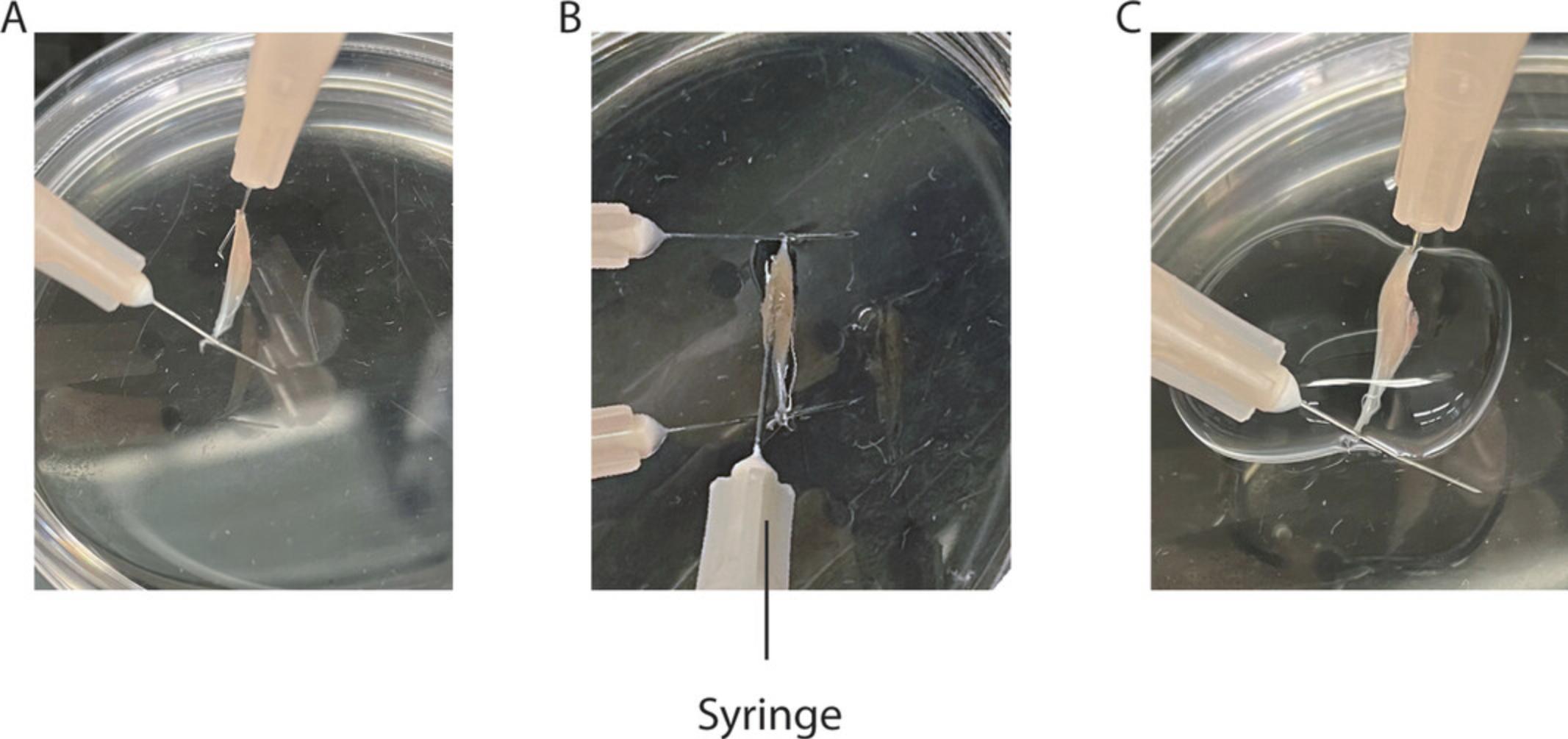
13.Insert a 27-G needle attached to a syringe filled with fixation buffer along the entire length of the muscle. Inject 200 μl of the solution while slowly pulling back the needle (Fig. 3B).
14.Add 1 ml fixation buffer to cover the muscle completely (Fig. 3C).
15.Incubate 5 min at room temperature.
16.Transfer the muscle into a well for washing (E.g., 12-well plate).
17.Wash the muscle two times, each time for 5 min with room temperature PBS.
18.Either store the muscle in PBS at 4°C or proceed with muscle fiber bundle isolation.
19.Isolate muscle fiber bundles.
-
Pin the EDL to the Sylgard plate on one of the tendons using 30-G needles in PBS (Fig.4A) and transfer the plate to an illuminated binocular microscope.
-
Use curved Dumont tweezers to longitudinally separate a piece of the EDL muscle (white arrow) with parts of both tendons (Fig.4B). Handle the tissue only on the tendons and avoid pinching of muscle fibers.
-
Store the remaining EDL muscle in PBS at 4°C.
-
Pin the separated piece of EDL to the Sylgard plate using one of the tendons (Fig.4C).
-
Carefully separate fibers longitudinally by using two curved Dumont tweezers without pinching, until only bundles of 3-4 fibers remain (Fig.4D). Keep the muscle fibers attached to the tendon that is pinned to the plate.
-
Using curved Dumont tweezers, transfer the MIFBs on the remaining tendon into a 1.7-ml horse serum−coated transparent reaction tube filled with PBS (Fig.4E).
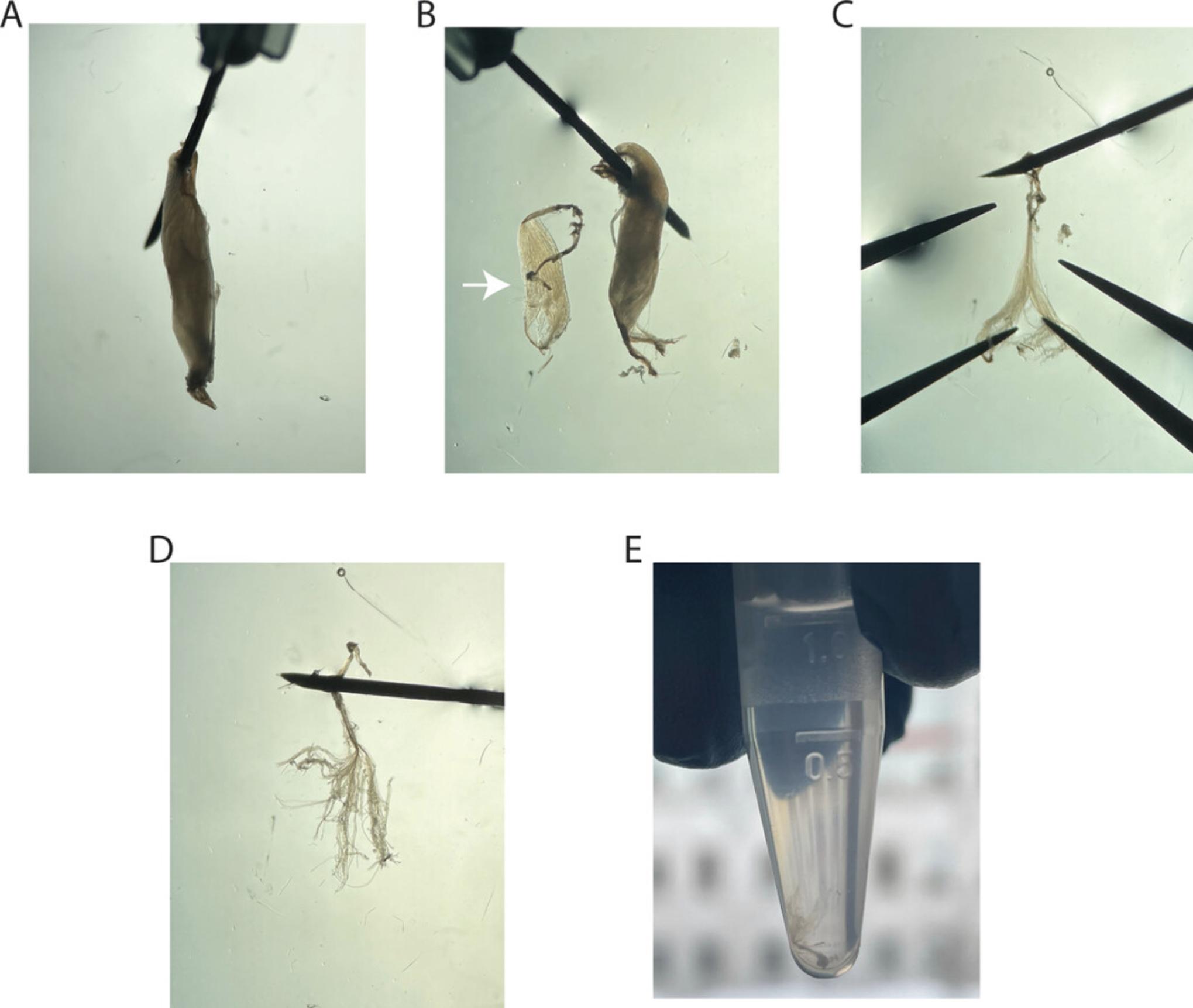
20.Either store the MIFBs at 4°C or continue with Basic Protocol 2: Immunofluorescence staining for MuSCs on fiber bundles.
Basic Protocol 2: IMMUNOFLUORESCENCE STAINING OF MuSCs ON FIBER BUNDLES
This protocol describes immunofluorescence staining of the basal lamina, MuSCs, endothelial cells, and FAPs on MIFBs using laminin, Pax7, M-cadherin, CD31, and PDGFRα antibodies. For removing buffers from the sample containing MIFBs, hold the reaction tube against a light source and use a one-piece transfer pipette with a thin opening hole. Remove as much buffer as possible, not leaving more than 100 μl in the tube. Work efficiently after the secondary antibody is applied and protect the sample from light. To add fresh buffer, tilt the sample and pipet the solution slowly against the wall of the reaction tube. Do not apply liquid directly to the MIFBs. Microscopy slides with mounted MIFBs can be stored at 4°C for up to 4 weeks.
Materials
-
MIFBs (Basic Protocol 1, step 20)
-
Phosphate-buffered saline (PBS), pH 7.4 (Bio Basic, cat. no. PD8117)
-
Permeabilization buffer (see recipe)
-
Blocking buffer (see recipe)
-
Fixation buffer (see recipe)
-
Dilution buffer (see recipe)
-
Primary antibodies:
- Rabbit anti-laminin (Sigma-Aldrich, cat. no. L9393, RRID:AB_477163)
- Mouse anti-Pax7 (DSHB Hybridoma concentrate, Pax7, RRID:AB_528428)
- Rabbit anti-M-cadherin (Cell Signaling, cat. no. D4B9L, RRID:AB_2799178)
- Rat anti-CD31 (Thermo Fisher Scientific, cat. no. 14-0311-85, RRID: AB_468931)
- Goat anti-PDGFRα (R&D systems, cat. no. BAF1062, RRID: AB_2162051)
-
Secondary antibodies:
- Donkey anti-mouse IgG Alexa Fluor 546 (Thermo Fisher Scientific, cat. no, A10036, RRID: AB_2534012)
- Donkey anti-rabbit IgG Alexa Fluor 488 (Thermo Fisher Scientific, cat. no. A-21206, RRID: AB_2535792)
- Goat anti-rat IgG Alexa Fluor 647 (Thermo Fisher Scientific, cat. no. A-21247, RRID: AB_141778)
- Donkey anti-goat IgG Alexa Fluor 647 (Thermo Fisher Scientific, cat. no. A-21447, RRID: AB_2535864)
-
Hoechst (Thermo Fisher Scientific, cat no. 62249)
-
Mowiol mounting medium (see recipe)
-
Microscope slides (Fisher Scientific, cat. no. 12-550-123)
-
Binocular microscope
-
Vannas scissors (Fine Science Tools Inc., cat. no. 15003-08)
-
Curved Dumont tweezers (Fine Science Tools Inc., cat. no. 11251-35)
-
Microscope cover slips (Fisher Scientific, cat. no. 12545F)
1.Remove PBS, not leaving more than 100 μl in the coated reaction tube with the MIFBs.
2.Permeabilize the samples with 500 μl permeabilization buffer for 30 min at room temperature.
3.Wash the MIFBs three times, each with 1 ml PBS for 5 min at room temperature.
4.Incubate with blocking solution for 1.5 hr at room temperature.
5.Apply 250 μl primary antibody in dilution buffer and incubate overnight at 4°C.
-
To stain for MuSC nuclei and laminin, use the following antibody dilutions:i.Mouse anti-Pax7 (1:100, 5-10 μg/ml).iiRabbit anti-laminin (1:1000, 0.5 μg/ml).
-
To stain for MuSC nuclei and morphology use the following antibody dilutions:i.Mouse anti-Pax7 (1:100, 5-10 μg/ml).ii.Rabbit anti-M-cadherin (1:200, 2 μg/ml).
-
To stain for MuSC nuclei and endothelial cells, use the following antibody dilutions:i.Mouse anti-Pax7 (1:100, 5-10 μg/ml).ii.Rat anti-CD31 (1:200, 2.5 μg/ml).
-
To stain for MuSC nuclei and FAPs use the following antibody dilutions:i.Mouse anti-Pax7 (1:100, 5-10 μg/ml).ii.Goat anti-PDGFRα (1:200, 1 μg/ml).
6.Wash the MIFBs five times with 1 ml PBS for 5 min at room temperature.
7.Incubate the MIFBs with 250 μl secondary antibodies and Hoechst (5 μg/ml) in dilution buffer for 1.5 hr at room temperature in the dark. Perform all subsequent steps under light protection.
1.For Pax7: Donkey anti-mouse IgG Alexa Fluor 546 (1:500, 4 μg/ml). 2.For laminin: Donkey anti-rabbit IgG Alexa Fluor 488 (1:500, 4 μg/ml). 3.For M-cadherin: Donkey anti-rabbit IgG Alexa Fluor 488 (1:500, 4 μg/ml). 4.For CD31: Goat anti-rat Alexa Fluor 647 (1:500, 4 μg/ml). 5.For PDGFRα: Donkey anti-goat Alexa Fluor 647 (1:500, 4 μg/ml).
8.Wash the MIFBs five times, each time with 1 ml PBS for 5 min at room temperature.
9.Spread one drop of Mowiol on a glass slide and carefully transfer the MIFBs to the slide on the tendon.
10.Using a binocular microscope, cut the remaining tendon connected to the MIFBs using Vannas micro scissors, and arrange the bundles using curved Dumont tweezers under the lowest possible light exposure.
11.Cover the slide with a cover slip. Avoid the introduction of air bubbles.
12.Let the Mowiol solidify overnight at room temperature.
13.Store the samples at 4°C until imaging.
Support Protocol: PREPARATION OF SYLGARD DISHES
This protocol describes the preparation of one 6-cm dish filled with Sylgard for the MIFB procedure. Sylgard dishes can be stored under dry conditions for several years.
Materials
- 6-cm dish (Sarstedt, cat. no. 83.3901)
- SYLGARD 182 Silicone Elastomer Kit (Dow, cat. no. 1673998)
- One-piece transfer pipette (Sigma-Aldrich, cat. no. Z350605)
1.Add 20 g of Sylgard 182 base to a 6-cm dish in a fume hood.
2.Slowly add 2 g of Sylgard 182 curing agent using a one-piece transfer pipette in a fume hood.
3.Use a one-piece transfer pipette to mix the solutions in the dish. Avoid the generation of air bubbles.
4.Let the dish solidify in the fume hood for 72 hr.
REAGENTS AND SOLUTIONS
Blocking buffer
5% BSA and 13 µg/ml Fab fragment goat anti-mouse IgG in phosphate-buffered saline (PBS), pH 7.4 (Jackson Immuno Research, cat. no. 115-007-003).
Dilution buffer
- 5% BSA in PBS (Bio Basic, cat. no. PD8117), pH 7.4. Store up to 1 week at 4°C.
Horse serum−coated reaction tubes, 1.7-ml
- Fill a 1.7-ml reaction tube (Axygen, cat. no. MCT-175-C) with horse serum (Wisent, cat. no. 065-150) and incubate for 5 min.
- Remove the serum and dry the tube for 5 min at room temperature.
- Store up to one day at 4°C.
Fixation buffer
- 4% paraformaldehyde (PFA) in phosphate-buffered saline (Bio Basic, cat. no. PD8117), pH 7.4. Store up to 4 weeks at 4°C or up to 1 year at −20°C.
Mowiol mounting medium
- 2.4 g Mowiol 4-88 (Sigma-Aldrich, cat. no. 81381-50G)
- 6 g glycerol (Thermo Fisher Scientific, cat. no. G31-1)
- 0.2 M Tris·HCl pH 8.5 (Thermo Fisher Scientific, cat. no. BP153-1)
- DABCO (Sigma-Aldrich, cat. no. 290734-100ML)
- Preparation
- Mix 2.4 g of Mowiol and 6 g of glycerol.
- Add 6 ml water.
- Incubate at room temperature for 5 hr.
- Add 12 ml 0.2 M Tris·HCl pH 8.5.
- Incubate at 50°C for 10 min.
- Spin down 15 min at 5000 × g.
- Add DABCO to a final concentration of 2.5%.
- Aliquot and store at −20°C for several years or 4°C 1-2 weeks.
Permeabilization buffer
- 0.1% Triton X-100 in PBS (Bio Basic, cat. no. PD8117), pH 7.4. Store up to 2 weeks at 4°C.
COMMENTARY
Background Information
Fiber bundles allow for visualization of MuSCs with intact niche components such as ECM and supportive cell types above the basal lamina, as well as the microvasculature. We observed that collagenase digestion used for single-fiber isolation leads to damage to the basal lamina, which may lead to detachment of ECM receptors expressed by MuSCs, contributing to their activation (Fig. 5A). In contrast, the basal lamina appears thicker and fully intact in MIFBs. Moreover, likely through the same mechanism, enzymatic digestion leads to the retraction of cytoskeletal protrusions of MuSCs during the isolation procedure (Fig. 5B). This observation supports the notion that MuSCs on EIFs already become primed for proliferation during enzymatic digestion. Thus, the fiber-bundle method is ideally suited for investigation of ECM interactions and the characterization of the cytoskeleton of MuSCs in bona fide quiescence.
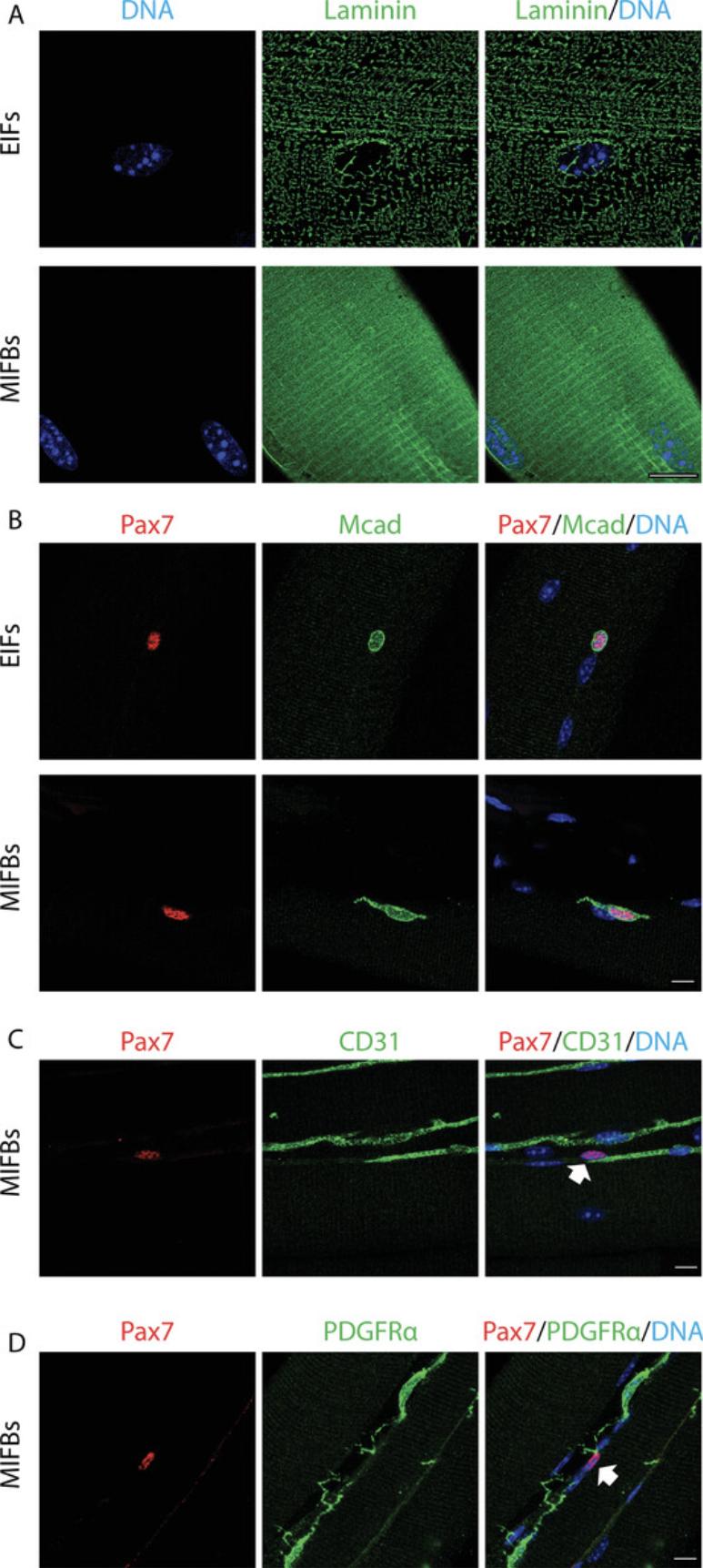
MuSC function is controlled by diverse niche cell types residing in the interstitial space (Bentzinger et al., 2013). Moreover, MuSCs are often closely localized to microvessels providing access to nutrients and oxygen as well as systemic and local signaling factors. In MIFBs, large portions of the microvasculature surrounding the basal lamina are preserved, and their close association with MuSCs can be visualized and analyzed (Fig. 5C). FAPs are major sources of ECM and paracrine regulatory signals for MuSCs in skeletal muscle. Using MIFBs, we observed that FAPs in the native niche have extensive cytoskeletal protrusions that often superimpose with MuSCs and likely have regulatory functions (Fig. 5D).
In contrast to the straightforward MIFB method, other techniques enabling the investigation of MuSCs in their endogenous niche without enzymatic digestion require extensive tissue clearance protocols and specialized microscopy techniques (Verma, Murkonda, Asakura, & Asakura, 2016; Williams et al., 2019). Importantly, muscle fiber bundles are also well suited for staining and analysis of the neuromuscular and myotendinous junction using appropriate fluorescent reagents. Moreover, the fiber bundle method may well be combined with RNA in situ visualization to address discrepancies between transcriptional and translational activities in quiescent and activated MuSCs (Crist, Montarras, & Buckingham, 2012).
Altogether, fiber bundles provide a novel method for investigating MuSC quiescence and the stem cell niche using standard immunofluorescence and microscopy techniques. In future studies, this technique will also enable the thorough investigation of transcriptional, translational, and post-translational characteristics of quiescent MuSCs and other niche cell types.
Critical Parameters
Special attention should be paid when handling unfixed EDL muscles. Only handle muscles on the tendons, as touching the fibers may damage them and lead to contraction. To ensure proper hardening, prepare your Sylgard dish at least 3 days before use.
Troubleshooting
Table 1 lists problems that may arise with these methods along with their possible causes and solutions.
Problem | Possible cause | Solution |
---|---|---|
High background in the Pax7 staining | Secondary anti−mouse IgG binds to endogenous antigens in the tissue | Use a more specific anti-mouse IgG1 secondary antibody |
Weak Pax7 signal | The Pax7 antibody is hybridoma derived and can vary in concentration | Use a higher Pax7 antibody concentration or enhance the signal using biotinylated secondary antibodies and Alexa Fluor−coupled streptavidin. |
Poor overall staining | Permeabilization was insufficient | Permeabilize longer |
Staining diffuse | Antibodies cross-react with unspecific antigens | Determine the background using isotype IgG controls and omission of the primary antibody. If necessary, use different antibodies. |
Double labeling is unspecific | Cross-reactivity of primary or secondary antibodies | Compare to single stainings of the respective antigens. If necessary, use different antibodies. |
Sample difficult to analyze | Teasing is insufficient and too many fibers overlap in the bundles | Tease smaller bundles |
Understanding Results
Depending on the objective of the experiment, MuSCs or other cell types located sideways or on top of the muscle fibers in the mounted sample are preferable for analysis. To avoid interference with background originating from the muscle fiber, quantifications requiring immunofluorescence intensity analysis are ideally performed with cells located sideways. To investigate morphological characteristics of MuSCs such as cytoskeletal protrusions, cells located on top of the muscle fiber are preferable.
In order to analyze distances between different cell types in the MuSC niche, nuclei should be located in the same z -plane. Thus, confocal microscopy is ideally suited for imaging of MIFBs. We demonstrate that MuSCs and FAPs or endothelial cells can be co-visualized using our protocol (Fig. 5C and 5D). Similarly, macrophages, neutrophils, or fibroblasts, among others, can readily be visualized using the appropriate markers. Moreover, we have successfully used the fiber bundle protocol with skeletal muscles ≥5 days post cardiotoxin injury when newly formed muscle fibers have been established and allow for teasing.
Time Considerations
The muscle fiber bundle isolation approximately takes 1 hr per animal. Immunofluorescence staining of the muscle fiber bundles takes 2 consecutive days. Preparation of the Sylgard dish takes 3 consecutive days.
Acknowledgments
The authors thank Markus A. Ruegg and Gabriela Bezakova who developed the basis of this protocol for neuromuscular junction analysis. C.F.B. is supported by the Canadian Institutes of Health Research (CIHR, PJT-162442), the Natural Sciences and Engineering Research Council of Canada (NSERC, RGPIN-2017-05490), the Fonds de Recherche du Québec—Santé (FRQS, Dossiers 296357, 34813, and 36789), the ThéCell Network (supported by the FRQS), the Canadian Stem Cell Network, and a research chair of the Centre de Recherche Médicale de l'Université de Sherbrooke (CRMUS). S.D. is supported by a fellowship from the FRQS, and S.C.S. is supported by a fellowship of the Centre de Recherche du Centre Hospitalier Universitaire de Sherbrooke (CRCHUS).
Author Contributions
S.D., S.C.S., and C.F.B adapted the MIFB method for visualization of the MuSC niche. All authors contributed to manuscript preparation.
Svenja C. Schüler : investigation, methodology, writing original draft, writing review and editing; Simon Dumontier : investigation, methodology, writing original draft, writing review and editing; Jonathan Rigaux : investigation, methodology, writing original draft, writing review and editing; C. Florian Bentzinger : conceptualization, funding acquisition, investigation, methodology, project administration, supervision, writing original draft, writing review and editing.
Conflict of Interest
The authors declare no conflict of interest.
Open Research
Data Availability Statement
Raw data are available upon request to the corresponding author.
Literature Cited
- Bentzinger, C. F., Wang, Y. X., Dumont, N. A., & Rudnicki, M. A. (2013). Cellular dynamics in the muscle satellite cell niche. EMBO Reports , 14(12), 1062–1072. doi: 10.1038/embor.2013.182.
- Bentzinger, C. F., Wang, Y. X., & Rudnicki, M. A. (2012). Building muscle: Molecular regulation of myogenesis. Cold Spring Harbor Perspectives in Biology , 4(2), a008342. doi: 10.1101/cshperspect.a008342.
- Bentzinger, C. F., Wang, Y. X., von Maltzahn, J., Soleimani, V. D., Yin, H., & Rudnicki, M. A. (2013). Fibronectin regulates Wnt7a signaling and satellite cell expansion. Cell Stem Cell , 12(1), 75–87. doi: 10.1016/j.stem.2012.09.015.
- Bischoff, R. (1986). Proliferation of muscle satellite cells on intact myofibers in culture. Developmental Biology , 115(1), 129–139. doi: 10.1016/0012-1606(86)90234-4.
- Crist, C. G., Montarras, D., & Buckingham, M. (2012). Muscle satellite cells are primed for myogenesis but maintain quiescence with sequestration of Myf5 mRNA targeted by microRNA-31 in mRNP granules. Cell Stem Cell , 11(1), 118–126. doi: 10.1016/j.stem.2012.03.011.
- Donovan, J., & Brown, P. (2006). Euthanasia. Current Protocols in Immunology , 73, 1.8.1–1.8.4. doi: 1002/0471142735.im0108s73.
- Joe, A. W., Yi, L., Natarajan, A., Le Grand, F., So, L., Wang, J., … Rossi, F. M. (2010). Muscle injury activates resident fibro/adipogenic progenitors that facilitate myogenesis. Nature Cell Biology , 12(2), 153–163. doi: 10.1038/ncb2015.
- Kuang, S., Kuroda, K., Le Grand, F., & Rudnicki, M. A. (2007). Asymmetric self-renewal and commitment of satellite stem cells in muscle. Cell , 129(5), 999–1010. doi: 10.1016/j.cell.2007.03.044.
- Le Grand, F., Jones, A. E., Seale, V., Scime, A., & Rudnicki, M. A. (2009). Wnt7a activates the planar cell polarity pathway to drive the symmetric expansion of satellite stem cells. Cell Stem Cell , 4(6), 535–547. doi: 10.1016/j.stem.2009.03.013.
- Lukjanenko, L., Karaz, S., Stuelsatz, P., Gurriaran-Rodriguez, U., Michaud, J., Dammone, G., … Feige, J. N. (2019). Aging disrupts muscle stem cell function by impairing matricellular WISP1 secretion from fibro-adipogenic progenitors. Cell Stem Cell , 24(3), 433–446.e437. doi: 10.1016/j.stem.2018.12.014.
- Machado, L., Esteves de Lima, J., Fabre, O., Proux, C., Legendre, R., Szegedi, A., … Mourikis, P. (2017). In situ fixation redefines quiescence and early activation of skeletal muscle stem cells. Cell Reports , 21(7), 1982–1993. doi: 10.1016/j.celrep.2017.10.080.
- Pasut, A., Jones, A. E., & Rudnicki, M. A. (2013). Isolation and culture of individual myofibers and their satellite cells from adult skeletal muscle. Journal of Visualized Experiments , 73, e50074. doi: 10.3791/50074.
- Pouliot, Y., Gravel, M., & Holland, P. C. (1994). Developmental regulation of M-cadherin in the terminal differentiation of skeletal myoblasts. Developmental Dynamics , 200(4), 305–312. doi: 10.1002/aja.1002000405.
- Schmidt, M., Schüler, S. C., Hüttner, S. S., von Eyss, B., & von Maltzahn, J. (2019). Adult stem cells at work: Regenerating skeletal muscle. Cellular and Molecular Life Sciences , 76(13), 2559–2570. doi: 10.1007/s00018-019-03093-6.
- Seale, P., Sabourin, L. A., Girgis-Gabardo, A., Mansouri, A., Gruss, P., & Rudnicki, M. A. (2000). Pax7 is required for the specification of myogenic satellite cells. Cell , 102(6), 777–786. doi: 10.1016/s0092-8674(00)00066-0.
- Urciuolo, A., Quarta, M., Morbidoni, V., Gattazzo, F., Molon, S., Grumati, P., … Bonaldo, P. (2013). Collagen VI regulates satellite cell self-renewal and muscle regeneration. Nature Communications , 4, 1964. doi: 10.1038/ncomms2964.
- van Mourik, J. A., Leeksma, O. C., Reinders, J. H., de Groot, P. G., & Zandbergen-Spaargaren, J. (1985). Vascular endothelial cells synthesize a plasma membrane protein indistinguishable from the platelet membrane glycoprotein IIa. Journal of Biological Chemistry , 260(20), 11300–11306. doi: 10.1016/S0021-9258(17)39180-9.
- van Velthoven, C. T. J., de Morree, A., Egner, I. M., Brett, J. O., & Rando, T. A. (2017). Transcriptional profiling of quiescent muscle stem cells in vivo. Cell Reports , 21(7), 1994–2004. doi: 10.1016/j.celrep.2017.10.037.
- Verma, M., Asakura, Y., Murakonda, B. S. R., Pengo, T., Latroche, C., Chazaud, B., … Asakura, A. (2018). Muscle satellite cell cross-talk with a vascular niche maintains quiescence via VEGF and notch signaling. Cell Stem Cell , 23(4), 530–543.e539. doi: 10.1016/j.stem.2018.09.007.
- Verma, M., Murkonda, B. S., Asakura, Y., & Asakura, A. (2016). Skeletal Muscle tissue clearing for LacZ and fluorescent reporters, and immunofluorescence staining. Methods in Molecular Biology , 1460, 129–140. doi: 10.1007/978-1-4939-3810-0_10.
- Williams, M. P. I., Rigon, M., Straka, T., Horner, S. J., Thiel, M., Gretz, N., … Rudolf, R. (2019). A novel optical tissue clearing protocol for mouse skeletal muscle to visualize endplates in their tissue context. Frontiers in Cellular Neuroscience , 13, 49. doi: 10.3389/fncel.2019.00049.
Citing Literature
Number of times cited according to CrossRef: 1
- Jingxian Xie, Marlene Davis Ekpo, Jian Xiao, Hongbin Zhao, Xiaoyong Bai, Yijie Liang, Guang Zhao, Dong Liu, Songwen Tan, Principles and Protocols For Post-Cryopreservation Quality Evaluation of Stem Cells in Novel Biomedicine, Frontiers in Pharmacology, 10.3389/fphar.2022.907943, 13 , (2022).