Histodenz Separation of Lysosomal Subpopulations for Analysis of Chaperone-mediated Autophagy
Calvin M. Burns, Calvin M. Burns, Richard A. Miller, Richard A. Miller, S. Joseph Endicott, S. Joseph Endicott
Abstract
Chaperone-mediated autophagy (CMA) is the most selective form of lysosomal proteolysis, in which proteins are individually selected for lysosomal degradation. CMA degradation targets bear a pentapeptide consensus motif that is recognized by the cytosolic chaperone HSPA8 (Hsc70), which participates in the trafficking of the target to the lysosomal surface. From there, it is translocated into the lysosomal lumen, independent of vesicle fusion, in a process dependent upon the lysosomal transmembrane protein LAMP2A. There are limited tools for studying CMA in whole cells and tissues, and many of the best techniques for studying CMA rely on the preparation of lysosome enriched fractions. Such experiments include (1) the in vitro evaluation of CMA substrate uptake activity, (2) the characterization of changes to lysosomal resident and CMA regulatory proteins, and (3) lysosomal targetomics, i.e., the use of quantitative proteomics to characterize lysosomal degradation targets. Previous studies using discontinuous metrizamide gradients have shown that a subpopulation of liver lysosomes is responsible for the majority of CMA activity (“CMA+”). These CMA+ lysosomes are low density and have higher levels of MTORC2 relative to the “CMA–” lysosomes, which are high density and have higher levels of MTORC1. Because of safety concerns surrounding metrizamide, however, this compound is difficult to obtain, and it is impractically expensive. Here, we have provided protocols for isolation of lysosomal subpopulations for CMA-related analyses from mouse liver using Histodenz, a safe and affordable alternative to metrizamide. Supplementary protocols show how to perform CMA activity assays with appropriate statistical analysis, and how to analyze for lysosomal breakage/membrane integrity. © 2024 The Authors. Current Protocols published by Wiley Periodicals LLC.
Basic Protocol : Isolation of lysosomal subpopulations from mouse liver using discontinuous Histodenz gradients
Alternate Protocol : Isolation of lysosomes from cultured cells using discontinuous Histodenz gradients
Support Protocol 1 : Verifying enrichment of lysosomal markers in lysosome-enriched fractions
Support Protocol 2 : Measuring in vitro uptake of CMA substrates
Support Protocol 3 : Measuring lysosomal membrane integrity by hexosaminidase assay
INTRODUCTION
Autophagy is the recycling of organelles, proteins, and other macromolecules through the lysosome. Autophagy was originally thought to be a non-selective bulk-recycling mechanism. However, we now understand that most forms of autophagy are highly selective, and that targeted degradation of intracellular cargo through the lysosome is required for cellular adaptations to stress, remodeling the cytoplasm during differentiation, and maintaining proteomic and metabolic homeostasis (Mizushima & Levine, 2010; Murrow & Debnath, 2013; Ryter et al., 2013). The three primary forms of autophagy present in all mammalian cell types are macroautophagy, microautophagy, and chaperone-mediated autophagy (CMA). Each of these has its own sophisticated selection mechanisms for the selection of degradation targets (Johansen & Lamark, 2014; Sahu et al., 2011).
CMA, which targets individual proteins for lysosomal degradation, is the most selective form of autophagy (Aniento et al., 1993; Cuervo et al., 1995). CMA target proteins contain a consensus motif resembling Lys-Phe-Glu-Arg-Gln (KFERQ), which is recognized by the cytosolic chaperone HSPA8 (Hsc70; Agarraberes & Dice, 2001; Chiang et al., 1989; Dice, 1990). HSPA8, along with other cytosolic chaperones, participates in the trafficking of the KFERQ-bearing target to the lysosomal surface, where it is translocated directly across the lysosomal membrane through an incompletely characterized mechanism that is dependent on LAMP2A (encoded by one of three splice variants of lamp2 ; Agarraberes & Dice, 2001; Bandyopadhyay et al., 2008; Cuervo & Dice, 1996).
Preparation of lysosome enriched fractions from cells or animal tissues can be useful for the evaluation of several aspects of lysosomal biology, including, but not limited to, (1) the in vitro evaluation of CMA substrate uptake activity, (2) the characterization of changes to lysosomal resident proteins, many of which regulate CMA, and (3) lysosomal targetomics, which is the use of quantitative proteomics to characterize differences in the lysosomal accumulation of degradation targets. Targetomics makes use of lysosome-enriched fractions prepared from mice treated with a protease inhibitor, so that lysosomal targets accumulate in the lysosomal lumen without being degraded.
Isolation of lysosome enriched fractions has been essential for understanding the basic biology of CMA (Arias et al., 2015; Cuervo et al., 1995), for characterizing the lysosomal targetome (Endicott et al., 2021; Hao et al., 2019; Kacal et al., 2021), and for characterizing the relationship between CMA and aging (Cuervo & Dice, 2000; Zhang, Zhang et al., 2023). These protocols serve as a starting point for researchers looking to isolate lysosomal subpopulations for studying CMA.
Previous studies using discontinuous metrizamide gradients have shown that a subpopulation of lysosomes isolated from the livers of mice or rats is responsible for the majority of CMA activity (“CMA+”) (Arias et al., 2015; Cuervo et al., 1997). These CMA+ lysosomes are low density and have higher levels of MTORC2 as compared to CMA– lysosomes, which are high density and have higher levels of MTORC1 (Arias et al., 2015). In this paper, we will refer to the low-density CMA+ lysosome subpopulation as the “light lysosome fraction” and the high-density CMA– lysosome subpopulation as the “heavy lysosome fraction.” The relative distribution of the two different MTOR complexes between the lysosomal subpopulations has been reported to change in response to fasting. Rats exposed to a short-term fast (6 hr) show equal amounts of MTORC1 complex marker RAPTOR in light and heavy lysosome fractions, while rats exposed to a long fast (48 hr) have low RAPTOR in the light fraction and high RAPTOR in the heavy fraction, comparable to rats fed ad libitum (Arias et al., 2015). Our recent publication using this method has also demonstrated that PTEN, a famous tumor suppressor and regulator of longevity, negatively regulates the lysosomal localization of AKT: mice with more PTEN expression have less lysosome-localized AKT than their wild-type siblings (Zhang, Burns et al., 2023).
Because of safety concerns surrounding metrizamide, this compound is difficult to obtain, and it is impractically expensive. Therefore, it is nearly impossible to replicate the exact methods used in the original experiments that separated the light and heavy lysosome subpopulations for characterization of CMA. Here, we describe a protocol using Histodenz, a safe and affordable alternative to metrizamide, to isolate two liver lysosome subpopulations of identical densities to those isolated in the foundational CMA studies that relied on metrizamide (Basic Protocol). We also provide instructions for preparing lysosome enriched fractions from cultured cells, using protocols in which metrizamide has been replaced with Histodenz (Alternate Protocol). After carrying out these protocols, researchers should verify that they have achieved appropriate enrichment and purity of lysosomes by western blotting (Support Protocol 1). These lysosome-enriched fractions can be used in CMA substrate uptake assays (Support Protocol 2). If lysosomes produced by these procedures are used for substrate uptake assays, then lysosomal membrane integrity should be verified by hexosaminidase leakage assays (Support Protocol 3). Altogether, these protocols will facilitate modern attempts to replicate and build upon the findings of the early CMA studies.
NOTE : All protocols involving animals must be reviewed and approved by the appropriate Animal Care and Use Committee and must follow regulations for the care and use of laboratory animals. Appropriate informed consent is necessary for obtaining and use of human study material.
Basic Protocol: ISOLATION OF LYSOSOMAL SUBPOPULATIONS FROM MOUSE LIVER, USING DISCONTINUOUS HISTODENZ GRADIENTS
This protocol, in which Histodenz is used as a separation medium, separates lysosomes from mouse liver into two subpopulations, each having identical density to the subpopulations derived by the previous metrizamide-based technique (Fig. 1A-C; Cuervo et al., 1997). The two lysosomal subpopulations enriched by this technique have the expected distribution of markers for MTORC1 (RAPTOR) and MTORC2 (RICTOR) (Fig. 1D and E; Arias et al., 2015). This method enriches for the lysosomal protein CTSB at 62 ± 19-fold for the light lysosome fraction and 85 ± 14-fold for the heavy lysosome fraction (Fig. 1E). This outperforms the previous metrizamide-based method, which enriched for CTSB at 37 ± 12-fold for fraction 1 and 37 ± 13-fold for fraction 2 (Cuervo et al., 1997).
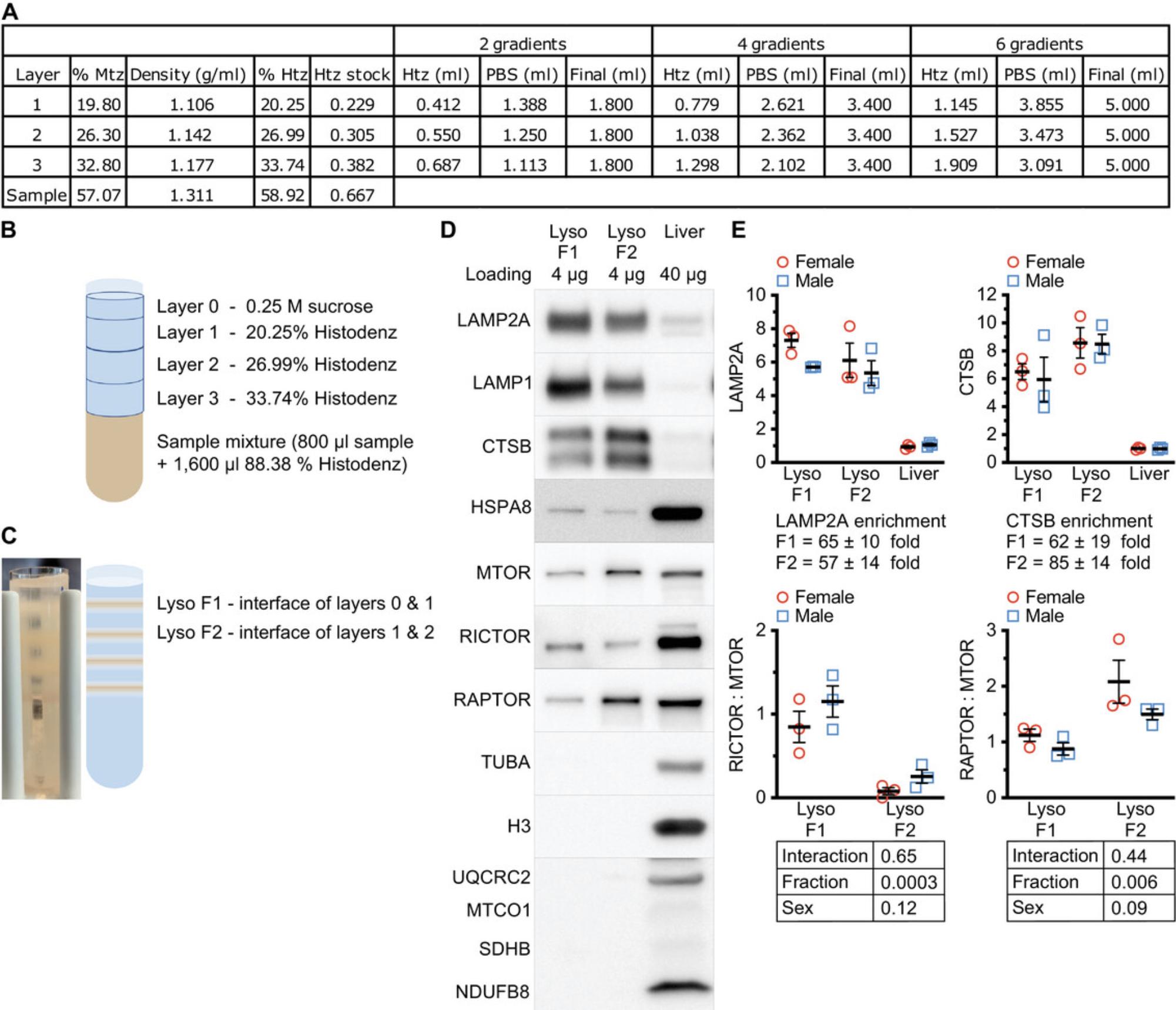
NOTE : The representative images presented in Figure 1 were produced using samples from a 4-month-old male mouse from the UM-HET3 stock (Jackson Laboratory, cat. no. 036603). All experiments conducted in this study were approved by the University of Michigan Institutional Animal Care and Use Committee (IACUC). Any future experiments using this protocol should receive local IACUC approval and comply with all national and institutional guidelines for the care and use of laboratory animals.
NOTE : All experiments conducted in an ultracentrifuge require rotational symmetry. For a swing-bucket rotor with 6 buckets, samples must be prepared in batches of 2, 3, 4, 6.
Materials
-
Phosphate-buffered saline (1× PBS), diluted from 10× stock (Gibco, cat. no. 7011-044)
-
88.38% Histodenz (Sigma, cat. no. D2158) stock solution (see recipe)
-
Dry ice (acquired from local supplier)
-
Liquid nitrogen (acquired from local supplier)
-
Adult (>3 months) mice, in numbers that will balance the ultracentrifuge (2, 3, 4, or 6)
-
0.25 M sucrose stock solution, pH 7.2-7.3 (see recipe)
-
MOPS/sucrose buffer (see recipe)
-
BCA Assay Kit (ThermoFisher, cat. no. 23227)
-
Milli-Q water machine (Millipore, cat. no. ZMQP60F01, or similar)
-
5-ml Ultra-Clear tubes (Beckman Coulter, cat. no. 344057)
-
Parafilm (Amcor, cat. no. PM-996)
-
Cold room with ∼6 square feet of counter space (you will need to brace your arms against the counter when making the gradients, so make sure the area is clear and wiped clean and that the counter is sturdy)
-
1.5- and 2-ml microcentrifuge tubes (USA Scientific, cat. nos. 1615-5510 and 1620-2700)
-
5-ml centrifuge tubes (USA Scientific, cat. nos. 4011-9487)
-
50-ml conical tubes (Fisher, cat. no. 05-539-9, or similar)
-
Dounce homogenizers (Wheaton, cat. no. 357538)
-
Swinging-bucket ultracentrifuge rotor: SW55Ti or SW50.1 (Beckman Coulter)
-
Ultracentrifuge (Beckman Coulter L70)
-
Styrofoam block or dissection board (repurpose the Styrofoam lid of a shipping box)
-
Disposable absorbent pads (Fisher, cat. no. 1420662)
-
Dissection tools: forceps, large scissors, small scissors, small spring-loaded scissors, dissection pins
-
5-ml test-tube rack (Endicott-Seymour, cat. no. 207)
-
Flat dry bath block (Stellar Scientific, cat. no. BS-HB-BSO, or similar)
-
Ice buckets (MidSci, cat. no. IPAN-2000, or similar)
-
Cardboard freezer boxes (Thermo Scientific, cat. no. 13-994-076, or similar)
-
Styrofoam cooler boxes (best to re-use a shipping box)
-
Liquid nitrogen vessels (Oxford Instruments, cat. no. 51-1625-0192)
-
1-ml syringes (Becton Dickinson cat. no. 309628) fitted with 27-G needles (Becton Dickinson cat. no. 305109)
-
−80°C freezer (ThermoFisher, cat. no. TSU600A, or similar), for sample storage
-
Tabletop microcentrifuge, refrigerated, capable of a 17,000 × g spin (ThermoFisher, cat. no. 75002463, or similar)
-
96-well plates (Fisher, cat. no. 12565501)
-
Microplate reader (BioTek Epoch2, 96-well format, or similar)
Experimental setup (perform the day before the main procedure)
1.In a 5-ml tube, dilute the 88.38% Histodenz stock solution in PBS to make the solutions that will become layers 1, 2, and 3 of the discontinuous gradients. 800 μl is required for each layer. Extra solution should be made to account for volume loss during pipetting. Figure 1A provides a quick reference on the relative volumes of Histodenz and PBS to use for each layer. Store the solutions at 4°C.
2.Add 1600 μl of 88.38% Histodenz stock to the bottom of the Ultra-Clear tubes that will be used the next day. Put Parafilm over the top to prevent evaporation. Store at 4°C.
3.Check that the centrifuge rotor assembly is in the cold room or refrigerator.
4.Set up the dissection area by wrapping the dissection board in a disposable absorbent pad and using dissection pins to hold the absorbent pad in place. Lay absorbent pads on the benchtop to prevent messes. Lay out the tools you will use the next morning.
5.Optional : Under most conditions, to examine autophagy, it is better to isolate lysosomes from mice that have been fasted for 18 hr or more. To fast the mice, place them into a clean cage with no food, and label the cage in accordance with your institutional regulations. Make sure that the mice have the appropriate environmental enrichment and access to fresh water.
Preparation of lysosome-enriched fractions from mouse liver
6.Set up the work area:
-
Place plastic water-bath racks into the ice. Place all needed tubes and dounce homogenizers in the ice-embedded water bath racks to keep them orderly.
-
Embed the dry bath block in ice, to use as a dissection surface.
-
Prepare a Styrofoam cooler box with dry ice on the bottom. Place a cardboard freezer box on the dry ice.
-
Fill a liquid nitrogen vessel with liquid nitrogen.
-
Assemble 1-ml syringes with 27-G needles. Do not uncap the needles.
7.Euthanize the mouse as quickly and humanely as possible, according to your institution's IACUC guidelines.
8.Working quickly, use a syringe fitted with a 27-G needle to perform a cardiac puncture and exsanguinate the mouse (this reduces blood contamination in the liver samples).
9.Working as quickly as possible, dissect out the liver and place it on the dry bath block that is embedded in ice.
10.Place ∼200 mg of liver tissue in a 1.5-ml tube to use later for making whole-liver lysate. Snap freeze tube in liquid nitrogen, and then transfer it to the sample box on dry ice (you can transfer it to the −80°C freezer at your convenience during one of the centrifuge spins, below).
11.Cut the remainder of the liver (no more than 750 mg) into coarse pieces and transfer them into 2-ml microcentrifuge tube. Perform all subsequent steps on ice, wherever possible.
12.Add 1 ml ice-cold 0.25 M sucrose to the liver pieces. Place the end of small dissection scissors into the tube and dice the liver in the sucrose solution as finely as possible.
13.Transfer the sucrose solution containing the minced liver into a Wheaton dounce homogenizer.
14.Use ∼33 strokes with the “LOOSE” pestle (0.089- to 0.14-mm gap) to homogenize the tissue (on ice).
15.Transfer the homogenate to a 2-ml centrifuge tube and adjust the volume to 2 ml with 0.25 M sucrose. Centrifuge 3 min at 6800 × g , 4°C.
16.Collect the supernatant, carefully avoiding the pellet, and transfer to a clean tube on ice.
17.Centrifuge the supernatant from the previous step for 10 min at 17,000 × g , 4°C.
18.Keep the pellet. This pellet contains the lysosomes and mitochondria. Discard the supernatant.
19.Resuspend the pellet in 600 μl ice-cold 0.25 M sucrose (be gentle so as not to break the lysosomes). Adjust the volume to 800 μl with 0.25 M sucrose. Add the sample to the Ultra-Clear tubes containing 1600 μl of 88.38% Histodenz (from step 2), and mix by pipetting up and down gently several times.
20.Take the samples to the cold room and repeat steps 15 and 16 there.
21.Place the Ultra-Clear tubes into a test tube rack that will not obscure your view of the entire length of the tube. Load the gradient fractions over the sample in the Ultra-Clear tube from bottom to top as follows (Fig. 1B):
-
0.8 ml 33.74% Histodenz
-
0.8 ml 26.99% Histodenz
-
0.8 ml 20.25% Histodenz
-
Add 0.25 M sucrose to the top until tube is full to within 3 mm of the top.
Gradient layers should be loaded slowly and carefully. You should be able to observe a sharp interface between each layer.
22.Place Ultra-Clear tubes into the swinging buckets (which should be precooled to 4°C). Weigh the buckets to make sure that the centrifuge rotor assembly will be balanced.
23.Take the samples to the ultracentrifuge, assemble the swinging buckets onto rotor, and load the rotor into the ultracentrifuge.
24.For an SW50.1Ti rotor assembly, set the centrifuge to 39,000 rpm and spin for 2 hr at 4°C.
25.Gently remove the rotor to avoid disrupting the gradients. Being very careful to keep the swinging buckets vertical, remove them from the rotor assembly and place them in their rack.
26.The light lysosome fraction will form an opaque band at the 20.25% Histodenz/0.25 M sucrose interface (Lyso F1 in Fig. 1C). The heavy lysosome fraction will form an opaque band at 26.99% Histodenz/20.25% Histodenz interface (Lyso F2 in Fig. 1C). Using a 200-μl (P200) pipet, carefully remove the lysosome bands to prelabeled 2-ml tubes.
27.Dilute the lysosomes from the gradient with at least 3 vol ice-cold PBS.
28.Spin the samples in a tabletop microcentrifuge for 30 min at 17,000 × g , 4°C. The lysosomes should pellet. Discard the supernatant.
29.Wash the pellet with 500 μl of whatever buffer is appropriate for your next assay (if in doubt, use MOPS/sucrose buffer). Spin 10 min at 16,000 × g , 4°C.
30.Discard the supernatant and resuspend the lysosomes in the appropriate assay buffer (if proceeding to substrate uptake assays in Support Protocols 2 and 3, use MOPS/sucrose buffer).
31.Following the manufacturer's instructions, perform a Pierce BCA protein quantification assay, using the 96-well-plate format suitable for use in the BioTek Epoch2 microplate reader.
32.Adjust the volumes of the samples so that the protein concentration of each sample is the same; 2 μg/μl is ideal. You will need at least 1 μg/μl for downstream assays. Keep the lysosomes on ice.
33.If analyzing CMA substrate uptake, or measuring lysosome membrane integrity, do not freeze or store the lysosomes; proceed immediately to Support Protocols 2 and 3.Otherwise, lysosomes should be stored at −80°C for up to 1 year.
Alternate Protocol: ISOLATION OF LYSOSOMES FROM CULTURED CELLS USING DISCONTINUOUS HISTODENZ GRADIENTS
Lysosome-enriched fractions prepared from cultured cells do not resolve into populations at the same densities as lysosomes prepared from liver, and studies using lysosomes from both liver and cultured cells typically use two different isolation methods (Arias et al., 2015). The most commonly used density centrifugation method for preparing lysosome-enriched fractions from cultured cells relies on a sequential gradient separation, where both gradients contain layers made with metrizamide. This method is based on a protocol by Storrie & Madden (1990). However, when we evaluated this method with discontinuous gradients made from Histodenz (which were identical in density to the original metrizamide gradients), we found that the sequential gradient separation method did not outperform the single-gradient method used in Basic Protocol (Fig. 2).
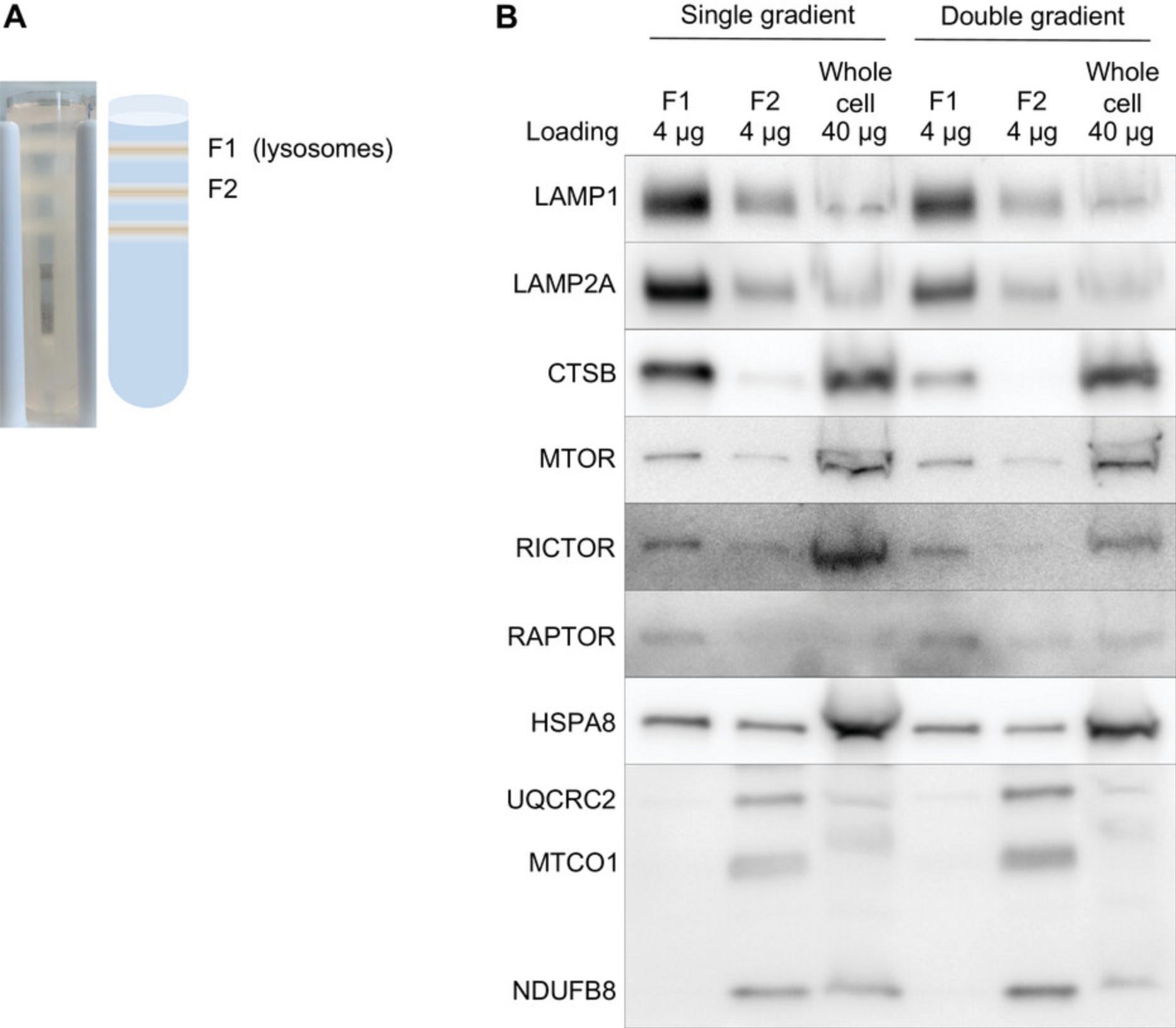
The dounce homogenization method used to lyse cells from dissected liver does not produce sufficient cell lysis when using cultured AML12 cells (normal mouse hepatocytes; data not shown). This protocol relies on nitrogen cavitation for detergent-free cell lysis. The optimal pressure for cell lysis can vary between cell types. It is advisable to empirically determine the optimal nitrogen pressure for cell lysis before starting this protocol (see Critical Parameters section).
Materials
-
1× Ca2+/Mg2+-free phosphate-buffered saline (PBS), diluted from 10× stock (Gibco, cat. no. 7011-044)
-
88.38% Histodenz (Sigma, cat. no. D2158) stock solution (see recipe)
-
At least four 175-ml (T175) flasks of AML12 cells at 90%-100% confluence for each experimental group (the number of experimental groups must balance an ultracentrifuge rotor—2, 3, 4, or 6)
-
Compressed nitrogen, at least 1000 psi (acquired from local supplier)
-
0.25 M sucrose stock solution, pH 7.2-7.3 (see recipe)
-
MOPS/sucrose buffer (see recipe)
-
BCA Assay Kit (ThermoFisher, cat. no. 23227)
-
Milli-Q water machine (Millipore, cat. no. ZMQP60F01, or similar)
-
5-ml centrifuge tubes (USA Scientific, cat. no. 4011-9487)
-
5-ml Ultra-Clear tubes (Beckman Coulter, cat. no. 344057)
-
Ice buckets (MidSci, cat. no. IPAN-2000, or similar)
-
Plastic wrap (Fisher, cat. no. 22-305654, or similar)
-
Cell scrapers (Fisher, cat. no. 08-100-241, or similar)
-
1.5- and 2-ml microcentrifuge tubes (USA Scientific, cat. nos. 1615-5510 and 1620-2700)
-
50-ml conical tubes (Fisher, cat. no. 05-539-9, or similar)
-
Cell culture centrifuge with a rotor suitable for 50-ml tubes (ThermoFisher, cat. no. 75004521, or similar)
-
Cell disruption vessel (45-ml capacity; Parr Instruments, cat. no. 04-731-50)
-
Teflon dounce homogenizers (instead of glass as used in the Basic Protocol; Grainger, cat. no. 23NE02, or similar)
-
Glass microscope slides and coverslips (for checking lysis efficiency)
-
Cell culture microscope (Nikon Eclipse TS100, equipped with a 10×/0.25 objective, Nikon, cat. no. MRP40102, or similar)
-
Tabletop microcentrifuge, refrigerated, capable of a 17,000 × g spin (ThermoFisher, cat. no. 75002463, or similar)
-
Cold room with ∼6 square feet of counter space (you will need to brace your arms against the counter when making the gradients, so make sure that the area is clear and wiped clean and that the counter is sturdy)
-
5-ml test-tube rack (Endicott-Seymour, cat. no. 207, or similar)
-
Swinging-bucket rotor: SW55Ti or SW50.1 (Beckman Coulter), precooled to 4°C
-
Ultracentrifuge (Beckman Coulter L70)
-
96-well plates (Fisher, cat. no. 12565501)
-
Probe sonicator (Fisher, model FB 120)
-
Microplate reader (BioTek Epoch2, 96-well format, or similar)
-
−80°C freezer for sample storage (ThermoFisher, cat. no. TSU600A, or similar)
Experimental setup (perform the day before the main procedure)
1.In a 5-ml tube, dilute the 88.38% Histodenz stock in PBS to make the solutions that will become layers 1, 2, and 3 of the discontinuous gradient. 800 μl are required for each layer. Make extra solution to account for volume loss during pipetting. Please refer to Figure 1A for a quick reference on the relative volumes of Histodenz and PBS to use for each layer. Store the solutions at 4°C.
2.Add 1600 μl of 88.38% Histodenz stock to the bottom of the Ultra-Clear tubes that will be used the next day. Put Parafilm over the top to prevent evaporation. Store at 4°C. Make sure that the ultracentrifuge rotor assembly is also at 4°C.
Experimental procedure
3.As quickly as possible, decant growth medium from the flasks, quickly rinse with 10 ml PBS, and decant again.
4.Add 10 ml PBS, and place flasks flat on ice for 10 min.
5.Using a rubber cell scraper, gently scrape the cells from the bottom of the flasks. Hold the flasks up to the light to make sure that no patches of cells remain adherent to the bottom.
6.Push the flasks into the ice at a 45° angle. Pipet the PBS over the bottom of the flasks to wash remaining cells loose. Pool the cells from the four flasks into a 50-ml conical tube.
7.Spin the 50-ml tubes in a cell culture centrifuge for 10 min at 600 × g , 4°C.
8.While step 7 is in progress, prepare your cell disruption vessel: make sure the bottom valve is tightly closed, wrap the bottom in plastic wrap (to keep it from getting wet), and embed it in a bucket of ice.
9.Remove the 50-ml tubes (from step 7) from the centrifuge. Decant the supernatants and resuspend the pellets in 1 ml of 0.25 M sucrose.
10.Load the first sample into the cell disruption vessel, and seal it tightly. Add nitrogen gas to 500 psi (note: the pressure will decrease at first, as the nitrogen dissolves in the sample, so add a little more at a time until it holds steady at 500 psi). Seal the input valve, disconnect from the nitrogen tank, and incubate for 7 min on ice.
11.Decant the sample slowly through the sample release valve, directly into the dounce homogenizer. The cells should be lysed at this point. 10-15 strokes with the pestle will strip the broken plasma membrane away from the nuclei, and release the lysosomes from the broken cells, increasing yield.
12.Place 10 μl of lysate onto a glass slide and add a coverslip. Examine on tissue culture microscope. You should see 80% or more free nuclei.
13.Take a 100-μl aliquot of whole-cell lysates for later analysis of lysosome enrichment and purity. Store on ice. Transfer the remaining homogenate to a 2-ml centrifuge tube and adjust the volume to 2 ml with 0.25 M sucrose.
14.Centrifuge samples for 3 min at 6800 × g , 4°C.
15.Collect the supernatant, carefully avoiding the pellet, and transfer to a clean tube.
16.Centrifuge the supernatant from the previous step for 10 min at 17,000 × g , 4°C.
17.Keep the pellet. This pellet contains the lysosomes and mitochondria. Discard the supernatant.
18.Resuspend the pellet in 600 μl of 0.25 M sucrose (be gentle so as not to break the lysosomes). Adjust the volume to 800 μl with 0.25 M sucrose. Add the sample to the Ultra-Clear tubes containing 1600 μl of 88.38% Histodenz (from step 2) and mix by pipetting up and down gently several times.
19.Take the samples to the cold room and perform steps 20 and 21 there.
20.Place the Ultra-Clear tubes into a test tube rack that will not obscure your view of the entire length of the tube. Load the tube from bottom to top as follows:
-
2.4 ml sample
-
0.8 ml 33.74% Histodenz
-
0.8 ml 26.99% Histodenz
-
0.8 ml 20.25% Histodenz
-
Add 0.25 M sucrose to the top until tube is full to within 3 mm of top.
Gradient layers should be loaded slowly and carefully. You should be able to observe a sharp interface between each layer.
21.Place Ultra-Clear tubes into the swinging buckets (which should be precooled to 4°C). Weigh the buckets to make sure that the centrifuge rotor assembly will be balanced.
22.Take the samples to the ultracentrifuge, assemble the swinging buckets onto rotor, and load the rotor into the ultracentrifuge. For an SW50.1Ti rotor assembly, set the centrifuge to 39,000 RPM and spin for 2 hr at 4°C.
23.While the lysosome samples are in the ultracentrifuge, prepare the whole-cell lysates set aside in step 13: vortex the samples thoroughly, and then sonicate them on the lowest amplitude setting, using 1-s pulses, until they are no longer viscous (usually 5 pulses). Store at −20°C until needed in step 29.
24.After the ultracentrifuge spin is complete, gently remove the rotor to avoid disrupting the gradients. Lysosomes will form an opaque band at the 20.25% Histodenz/0.25 M sucrose interface. Using a standard P200 micropipet, carefully remove the lysosome bands to labeled 2-ml tubes.
25.Dilute the lysosomes from the gradient with at least 3 vol ice-cold PBS.
26.Spin the samples in a tabletop microcentrifuge for 30 min at 17,000 × g , 4°C. The lysosomes should pellet. Discard the supernatant.
27.Wash the pellet with 500 μl of whatever buffer is appropriate for your next assay (if proceeding to substrate uptake assays, use MOPS/sucrose buffer). Spin 10 min at 16,000 × g , 4°C.
28.Discard the supernatant and resuspend the lysosomes in the appropriate assay buffer. Keep the lysosomes on ice
29.Follow the manufacturer's instructions, and perform a Pierce BCA protein quantification assay for the lysosome fraction and the whole-cell lysate, using the 96-well-plate format suitable for use in the BioTek Epoch2 microplate reader.
30.Adjust the volumes of the samples so that the protein concentration of each sample is the same; 2 μg/μl is ideal. You will need at least 1 μg/μl for downstream assays. Keep the lysosomes on ice.
31.If analyzing CMA substrate uptake or measuring lysosome membrane integrity, proceed immediately to Support Protocols 2 and 3.Otherwise, lysosomes should be stored at −80°C.
Support Protocol 1: VERIFYING ENRICHMENT OF LYSOSOMAL MARKERS IN LYSOSOME-ENRICHED FRACTIONS
The purpose of this protocol is to assess the relative enrichment (and purity) of lysosomal resident proteins in lysosomal fractions isolated from liver. First, this protocol describes how to prepare whole-liver lysates. Second, it describes how to prepare lysates of lysosome fractions. Third, it describes how to analyze lysosome fractions by western blotting.
Before beginning this protocol, users should have a basic understanding of how to operate a sonicator and how to perform western blots. The western blot protocol here is not meant to be comprehensive. Rather, it highlights important points that might differ from the protocols commonly used in other labs.
This protocol can also be used to assess the enrichment of lysosome resident proteins in lysosome fractions isolated from cultured cells. Simply substitute out the lysosome fraction samples from liver with those from cells, and replace the whole-liver lysates in the western blotting protocol below with whole-cell lysates.
NOTE : As much as possible, avoid using primary antibodies raised in a mouse host. The anti-mouse secondary antibody will react with the endogenous IgG heavy chain present in mouse serum (producing a band at 50 kDa). In this protocol, to evaluate for mitochondrial contamination, we used an OXPHOS antibody cocktail, where each antibody is raised in a mouse host. We do not show the band at ∼50 kDa because this is the molecular weight of both ATP5A and the endogenous IgG, making that band uninterpretable.
Materials
-
Liquid nitrogen (acquired from local supplier)
-
Dry ice (acquired from local supplier)
-
Liver tissue sample from Basic Protocol
-
Laemmli lysis buffer (see recipe)
-
Protease inhibitors (Sigma, cat. no. 11836153001)
-
PhosSTOP (Roche, cat. no. 04906845001)
-
70% ethanol (Volu-Sol, cat. no. VXB-128)
-
BCA Assay Kit (ThermoFisher, cat. no. 23227)
-
4× NuPage LDS sample loading buffer (Invitrogen, cat. no. NP0007)
-
2-Mercaptoethanol (Bio-Rad, cat. no. 161-0710)
-
Frozen lysosome samples from Basic Protocol
-
Lysis/loading buffer: NuPage LDS sample loading buffer, diluted to 1× in PBS, with 0.5% (v/v) 2-mercaptoethanol
-
15-well, 4-20% gradient gels (Bio-Rad, cat. no. 4561096, or similar)
-
Dual-color protein ladder (Bio-Rad, cat. no. 1610374)
-
Tris/glycine/SDS running buffer, diluted to 1× (Bio-Rad, cat. no. 1610732, or similar)
-
0.2-μm-pore-size PVDF transfer membrane (Bio-Rad, cat. no. 1620177, or similar)
-
100% methanol
-
Transfer buffer: Tris/glycine buffer (Bio-Rad, cat. no. 1610734), diluted to 1× in 80%/20% (v/v) water/methanol (Fisher, cat. no. A412-4, or similar)
-
Blocking buffer: 1× TBS, diluted from 10× stock (Bio-Rad, cat. no. 1706435), plus 0.1% (v/v) Tween (Sigma, cat. no. P1379) and 5% (w/v) dry milk powder (Research Products International, cat. no. M17200, or similar)
-
Wash buffer: 1× TBS + 0.1% (v/v) Tween, or similar
-
Primary antibodies: LAMP2A (AbCam, cat. no. ab125068), LAMP1 (AbCam, cat. no. ab24170), CTSB (Cell Signaling Technology, cat. no. 31718S), MTOR (Cell Signaling Technology, cat. no. 2972S), Total OXPHOS (AbCam, cat. no. MS604-300), RICTOR (Cell Signaling Technology, cat. no. 2214S), RAPTOR (Cell Signaling Technology, cat. no. 2280S), TUBA (Cell Signaling Technology, cat. no. 2144S), H3 (AbCam, cat. no. ab176842)
-
Secondary antibodies: HRP-conjugated goat pAb targeting rabbit IgG (AbCam, cat. no. ab6721) and HRP-conjugated goat pAb targeting mouse IgG (AbCam, cat. no. ab6789)
-
HRP substrate: EcoBright Femto (InnovSol, cat. no. EBFH100)
-
MAPA Temp-Ice 700 gloves (Magid, cat. no. M700410)
-
1.5- and 2-ml microcentrifuge tubes (USA Scientific, cat. nos. 1615-5510 and 1620-2700)
-
5-ml centrifuge tubes (USA Scientific, cat. no. 4011-9487)
-
Disposable absorbent pads (Fisher, cat. no. 1420662)
-
Liquid nitrogen vessels (Oxford Instruments, cat. no. 51-1625-0192, or similar)
-
Styrofoam cooler boxes (re-use a shipping box)
-
Tissue pulverizer with mallet (Cole-Parmer, cat. no. UX-36903-00)
-
Long forceps (Aven Tools, cat. no. 18432)
-
Brush (Fisher, cat. no. 03-670, or similar)
-
Laboratory spatulas (Cole-Parmer, cat. no. UX-06287-18, or similar)
-
Probe sonicator (Fisher, model FB 120)
-
Kimwipes (Kimtech, cat. no. 34155)
-
Tabletop microcentrifuge, refrigerated, capable of a 17,000 × g spin (ThermoFisher, cat. no. 75002463, or similar)
-
96-well plates (Fisher, cat. no. 12565501)
-
Microplate reader (BioTek Epoch2, 96-well format, or similar)
-
Dry bath (Fisher, cat. no. 11-718)
-
Gel electrophoresis and transfer apparatus (from Bio-Rad or similar)
-
Platform rocker (Corning, cat. no. 6705, or similar), placed inside a cold room at 4°C
-
Ice buckets (MidSci, cat. no. IPAN-2000, or similar)
-
Razors (Personna, cat. no. 94-120-71)
-
−80°C freezer for sample storage (ThermoFisher, cat. no. TSU600A, or similar)
-
Orbital shaker (Corning, cat. no. 6780, or similar)
-
GE Healthcare ImageQuant LAS 4000, using the manufacturer's ImageQuant LAS 4000 software
-
FIJI/ImageJ (version 2.3.0/1.53f or later)
Preparation of whole-liver lysates for western blotting
1.Put on the protective temp-ice gloves.
2.Set up the work area:
-
Label at least four 1.5-ml tubes with the name of each sample.
-
Lay a disposable absorbent pad over the work area
-
Decant liquid nitrogen from the holding tank into two liquid nitrogen vessels.
-
Cover the bottom of a Styrofoam cooler with dry ice.
-
Place the cardboard storage box with the samples on the dry ice, and carefully pour a small amount of liquid nitrogen over the samples.
-
Add two empty prelabeled 1.5-ml tubes for each sample into the box with the liquid nitrogen to precool. Leave the lids open. The hinges will become fragile when cold, so avoid unnecessary opening and closing.
-
Submerge the tissue pulverizer assembly in liquid nitrogen, and wait for the temperature to equilibrate.
3.When the tissue pulverizer has reached liquid nitrogen temperature, use the long forceps to lift it from the liquid nitrogen by the handle, and place it on the absorbent pad. Remove the pestle and set it to the side.
4.Working quickly, place the liver tissue sample in the mortar section of the pulverizer, and place the pestle back into the mortar.
5.Tap the top of the pestle with the provided mallet three times. Slightly lift and rotate the pestle one quarter turn, then tap it with the mallet three more times.
6.Using the brush, wipe down the bottom of the pestle and the sides of the mortar to accumulate all of the tissue powder in the bottom. Very carefully brush the tissue powder into the appropriate prelabeled, prechilled tube.
7.Repeat steps 1-6 for all samples.
8.From each powdered sample, take an aliquot of ∼100 μg (OK to estimate this by eye).
-
Working quickly, pick up a tube containing the sample and an empty prelabeled, prechilled 1.5-ml tube. Uncap them and hold them side by side between the index finger and middle finger.
-
Dunk the end of the small laboratory spatula in liquid nitrogen to cool it.
-
Scoop out a small amount of tissue powder and add it to the empty tube. You are aiming to fill it to the line indicating 100-μl volume.
-
Cap both tubes and return them to the box on dry ice.
-
Repeat for all samples.
9.Take a 10-ml aliquot of the homemade Laemmli lysis buffer, and add one protease inhibitor tablet and one PhosSTOP tablet. Pipet up and down to dissolve them.
10.Place the liver samples with the 100 μg of powder in a tube rack at room temperature. Add 400 μl Laemmli lysis buffer.
11.Set the sonicator to 50% power, with 10 pulses of 1 s on and 1 s off.
12.Place the tube over the tip of the sonicator probe until the probe is near the bottom of the tube (but not touching it). Sonicate the sample until it is homogenous, without any chunks of tissue.
13.Spin the sample for 30 min at 17,000 × g , 4°C.
14.Remove the samples from the centrifuge. There will be a layer of fat at the top of the sample, a transparent layer of amber or brown color in the middle, and a small pellet of insoluble material at the bottom. The middle layer contains the soluble proteins. Using a pipet, avoiding the fat and insoluble pellet, carefully remove 250 μl of the middle layer to a new tube. Repeat for each sample.
15.Follow the manufacturer's instructions and perform a Pierce BCA protein quantification assay, using the 96-well-plate format suitable for use in the BioTek Epoch2 microplate reader.
16.Adjust the volume of each sample so that the protein concentration is 6667 μg/μl.
17.Take a 150-μl aliquot of each sample and mix with 50 μl of 4× NuPage LDS sample loading buffer in a clean 1.5-ml tube. Add 2 μl of 2-mercaptoethanol.
18.Using the dry bath, heat the samples to 95°C for 5 min.
19.Cool the samples to room temperature and briefly spin in a centrifuge to collect condensation from the top of the tube.
Preparation of lysosome lysates for western blotting
20.Thaw lysosome samples on ice, in the ice bucket. Take a 30-μl aliquot of each lysosome sample and mix with 10 μl of 4× NuPage LDS sample loading buffer in a clean 1.5-ml tube. Add 0.4 μl of 2-mercaptoethanol.
21.Using the dry bath, heat the samples to 95°C for 5 min.
22.Cool the samples to room temperature and briefly spin in a centrifuge to collect condensation from the top of the tube.
Western blotting
23.Following the manufacturer's instructions, set up the Bio-Rad SDS-PAGE apparatus with 15-well, 4%-20% gradient gels. Fill the central chamber with the manufacturer's recommended Tris/glycine/SDS running buffer, diluted to 1×.
24.Load the gel with 6 μl of the dual-color protein ladder between sets of samples. Load 4 μg of lysosomal proteins and 40 μg of whole-liver lysate (Fig. 1D).
25.Fill the outer chamber with 1× Tris/glycine/SDS running buffer.
26.Run the gel for ∼90 min at 100 V, making sure that the dye front does not run off the end of the gel.
27.While the gel is running, dip the transfer membrane in 100% methanol, and then place it in transfer buffer and allow to equilibrate until it no longer floats.
28.Set up a transfer following the manufacturer's instructions for a Bio-Rad mini-transfer apparatus. Use all recommended buffers. Embed the transfer apparatus in ice and run for 2 hr at 70 V.
29.After the transfer, rinse membranes once with water. Place in blocking buffer for 20 min on the rotating platform.
30.Cut membranes to appropriate sizes, using a razor and straight edge, and incubate overnight at 4°C on the platform rocker in blocking buffer containing the primary antibodies, as shown in Fig. 1D.
Recommendations for primary antibody dilutions are :
LAMP2A | 1:5,000 |
LAMP1 | 1:5,000 |
CTSB | 1:1,000 |
OXPHOS | 1:5,000 |
MTOR | 1:500 |
RICTOR | 1:250 |
RAPTOR | 1:500 |
TUBA | 1:1,000 |
H3 | 1:100,000 |
31.Wash membranes once for 5 min in wash buffer.
32.Wash for 90 min in blocking buffer containing the appropriate HRP-conjugated secondary antibody (diluted 1:5000) on the orbital shaker.
33.Quickly rinse membranes with water twice and wash once for 5 min in wash buffer.
34.Apply the EcoBright Femto substrate directly to the membranes, and after 5 s, carefully blot off excess liquid with a paper towel.
35.Image membranes with a GE Healthcare ImageQuant LAS 4000, using the manufacturer's ImageQuant LAS 4000 software.
36.Quantify the band intensities using the “Analyze Gels” function of FIJI/ImageJ (version 2.3.0/1.53f or later).
37.Calculate relative enrichment. Do not forget to take difference in protein loading between the lysosome fractions and the whole-cell lysates into account.
Support Protocol 2: MEASURING IN VITRO UPTAKE OF CMA SUBSTRATES
Measuring the uptake of CMA-susceptible proteins by purified lysosomes is the oldest method for studying CMA (Aniento et al., 1993; Cuervo et al., 1994, 1995, 1997; Salvador et al., 2000). However, several variations on the design of this assay have been used over the last three decades of CMA research. The assay described here was chosen because of its relatively straightforward procedure and interpretation, and because it is more commonly used in modern CMA papers (Arias et al., 2015; Endicott, Boynton et al., 2020; Endicott, Ziemba et al., 2020). All samples tested by this protocol should also be evaluated for lysosome membrane integrity (see Support Protocol 3), in parallel to the analysis described here. This method uses recombinant MAPT as a CMA substrate. However, other substrates, such as GAPDH or SNCA, can be substituted for MAPT. This assay requires freshly prepared (never frozen) lysosome samples. This protocol should be performed immediately following either the Basic Protocol or the Alternate Protocol.
Materials
-
1.33× MAPT uptake assay buffer (see recipe)
-
1.33× blank uptake assay buffer (see recipe)
-
Protease inhibitors
-
MOPS/sucrose buffer (see recipe)
-
Fresh lysosome samples from Basic Protocol or Alternate Protocol
-
Lysis/loading buffer: NuPage LDS sample loading (Invitrogen, cat. no. NP0007) buffer diluted to 1× in 1× PBS (Sigma, cat. no. 11836153001), with 1% (v/v) 2-mercaptoethanol (Bio-Rad, cat. no. 161-0710)
-
Antibodies: LAMP2A (AbCam, cat. no. ab125068), MAPT (Cell Signaling Technology, cat. no. 46687S)
-
Milli-Q water machine (Millipore, cat. no. ZMQP60F01, or similar)
-
Floating tube rack (ThermoFisher, cat. no. 5974-0404, or similar)
-
Ice buckets (MidSci, cat. no. IPAN-2000, or similar)
-
1.5- and 2-ml microcentrifuge tubes (USA Scientific, cat. nos. 1615-5510 and 1620-2700)
-
5-ml centrifuge tubes (USA Scientific, cat. no. 4011-9487)
-
Tabletop microcentrifuge, refrigerated, capable of a 17,000 × g spin (ThermoFisher, cat. no. 75002463, or similar)
-
IsoTemp water bath (Fisher, cat. no. FTN#155462) at 37°C
-
−80°C freezer for sample storage (ThermoFisher, cat. no. TSU600A, or similar)
-
ImageJ software
Experimental setup (perform during 2-hr ultracentrifuge spin of the gradients in the Basic or Alternate Protocol)
1.Place a floating tube rack on ice. Label 1.5-ml microcentrifuge tubes for each experimental condition and sample, and place them in the tube rack on ice. There are three conditions for each sample: (1) MAPT + protease inhibitor (PI), (2) MAPT, (3) blank (Fig. 3A).
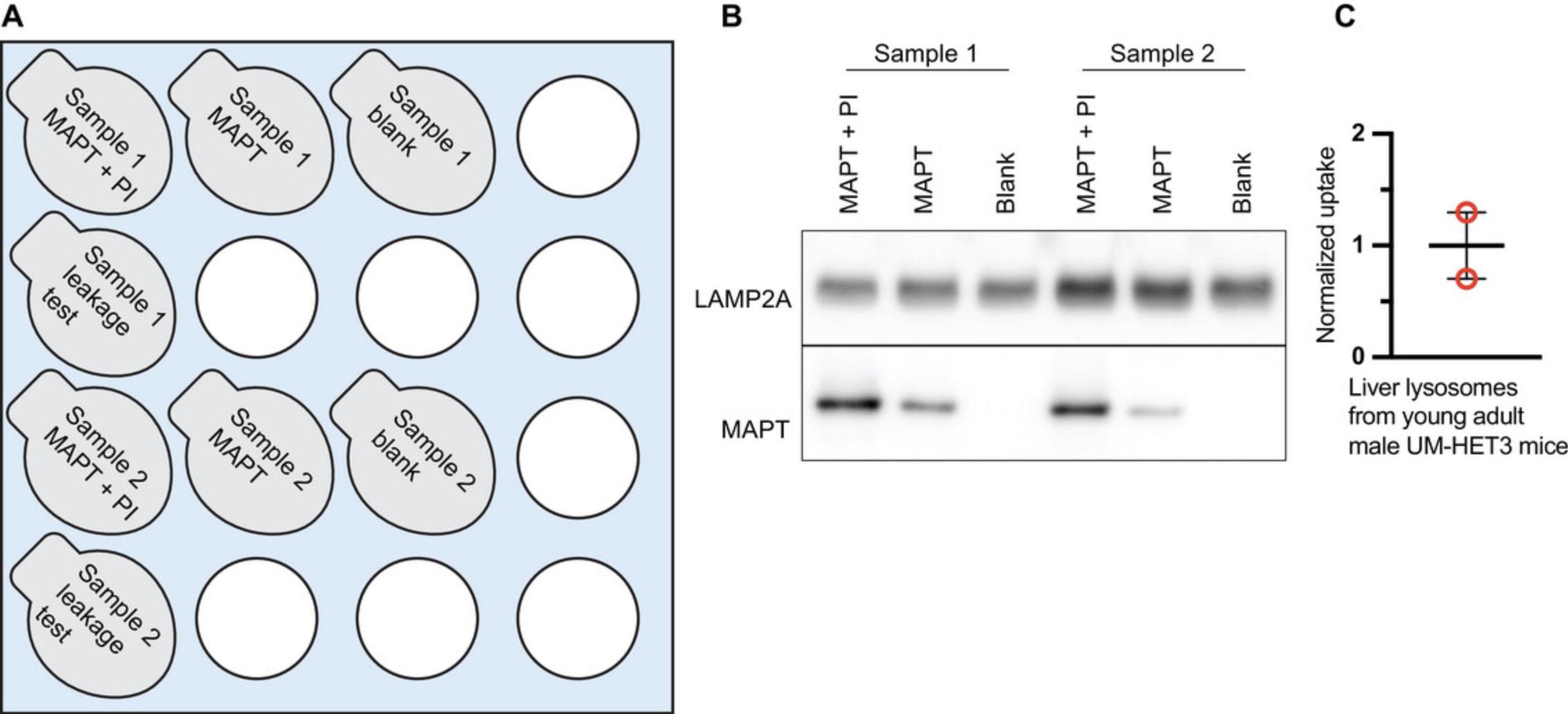
2.Add 30 μl of MAPT uptake assay buffer or blank uptake assay buffer to each tube, as appropriate (Fig. 3A).
3.Dissolve a protease inhibitor tablet in 500 μl MOPS/sucrose buffer. Add 5 μl of the solution to the tubes labeled “+ protease inhibitor.”
Experimental procedure—in vitro substrate uptake
4.Add 10 μl of freshly prepared lysosomes (concentration 1-2 μg/μl) to each of the three tubes labeled for each sample. Mix gently by pipetting up and down two or three times.
5.Take the ice bucket with the samples to the 37°C water bath. Place the floating tube rack in the bath and start the timer for 20 min.
6.After 20 min, immediately remove the floating tube rack from the water bath and return it to ice. Spin the samples in the tabletop microcentrifuge for 15 min at 16,000 × g , 4°C.
7.Carefully remove the samples and place them on ice—avoid disturbing the pellet. Carefully pipet off the supernatant, and discard it.
8.Gently resuspend each pellet in 40 μl MOPS/sucrose buffer.
9.Return to the 37°C water bath. Place the floating tube rack in the bath and start a timer for 10 min.
10.After 10 min, immediately remove the floating tube rack from the water bath and return it to ice. Spin the samples in the tabletop microcentrifuge for 15 min at 16,000 × g , 4°C.
11.Carefully place the samples on ice and remove the supernatant. The pellet can be frozen or resuspended directly in lysis/loading buffer (NuPage LDS sample loading buffer, diluted to 1× in PBS, with 0.5% (v/v) 2-mercaptoethanol).
Experimental procedure—evaluation of substrate uptake
12.Perform an analysis of the samples by standard western blotting procedures (see Support Protocol 1, if necessary). Blot for MAPT. Blot for LAMP2A as a loading control (Fig. 3B).
13.Quantify the band intensities using ImageJ.
14.Divide MAPT band intensity by the corresponding LAMP2A intensity to account for differences in loading of lysosomes.
15.Calculate uptake using loading-adjusted values from step 14.
16.Perform a plotting adjustment (normalization), to set the mean of the control to a value of “1,” and perform statistical analyses appropriate for your experimental setup.
Support Protocol 3: MEASURING LYSOSOMAL MEMBRANE INTEGRITY BY HEXOSAMINIDASE ASSAY
If the lysosomes are being used to assess lysosomal substrate uptake (i.e., chaperone-mediated autophagy), then it is necessary to verify that the lysosomal membrane is sufficiently intact to measure the internalization of substrate proteins. In this case, the hexosaminidase assay should be conducted in parallel to the substrate uptake assay (see Support Protocol 2). This method is only meant to evaluate freshly prepared lysosomal samples that have been kept on ice leading up to their use in biochemical experiments.
Additional Materials (also see Support Protocol 2)
- Colorimetric hexosaminidase buffer (see recipe)
- 96-well plates (Fisher, cat. no. 12565501)
- Microplate reader (BioTek Epoch2, 96-well format, or similar)
Experimental setup (perform during 2-hr ultracentrifuge spin of the gradients in the Basic or Alternate Protocol)
1.While setting up the CMA substrate uptake assay (in Support Protocol 2, step 1), place an extra prelabeled tube for each sample in the floating tube rack on ice, as indicated (Fig. 3A).
2.Add 30 μl blank uptake assay buffer to each tube.
Experimental procedure—perform in parallel with Support Protocol 2
3.Add 10 μl freshly prepared lysosomes (concentration 1 μg/μl) to each tube labeled for each sample. Mix gently by pipetting up and down two or three times.
4.Process samples as described in steps 5 and 6 of Support Protocol 2:
-
Take the ice bucket with the samples to the 37°C water bath. Place the floating tube rack in the bath and start the timer for 20 min.
-
After 20 min, immediately remove the floating tube rack from the water bath and return it to ice. Centrifuge the samples in the tabletop microcentrifuge for 15 min 16,000 ×g, 4°C.
5.Carefully remove the samples and place on ice—avoid disturbing the pellet. Carefully pipet off the supernatant and add it to a clean tube. Keep both the pellet and the supernatant.
6.Gently resuspend the pellets from step 5 in 50 μl of MOPS/sucrose buffer (add protease inhibitors to the MOPS/sucrose used to wash the samples that were previously treated with protease inhibitors).
7.Add 10 μl MOPS/sucrose buffer to each supernatant from step 5, bringing their volumes up to 50 μl.
8.Add 50 μl colorimetric hexosaminidase buffer to each sample (both pellet and supernatants).
9.Place the samples in a separate floating tube rack, and leave in the 37°C water bath overnight.
Experimental procedure—perform the next morning
10.Centrifuge the samples in the tabletop microcentrifuge for 15 min at 16,000 × g , 4°C.
11.Take the soluble portions of each sample and transfer them to a 96-well plate
12.Take the 96-well plate to the plate reader to measure absorbance at 405 nm.
13.Calculate the percentage of broken lysosomes.
14.If a sample has >10% broken lysosomes, exclude it from any other analyses.
REAGENTS AND SOLUTIONS
Blank uptake assay buffer, 1.33×
- 1.5 M sucrose stock (see recipe)
- 100 mM MOPS (Sigma, cat. no. M1254) stock, pH 7.2 (see recipe)
- 100 mM ATP (Sigma, cat. no. A2383) stock solution
- Recombinant HSPA8 (AbCam, cat. no. 78431)
- MAPT/Tau (Sino Biological, cat. no. 10058-H07E)
- Combine each component in Milli-Q water to final concentrations of 300 mM sucrose, 10 mM MOPS, 10 mM ATP, and 0.01 μg/μl HSPA8.
- Prepare fresh before each use. Keep on ice.
Colorimetric hexosaminidase buffer, 2×
Dissolve 1 tablet phosphate citrate (Sigma, cat. no. P4809) in 20 ml Milli-Q water, adjust the pH to 4.7, and then adjust the volume to 25 ml (final phosphate citrate concentration should be 200 mM). Add and dissolve in 4-nitrophenyl N -acetyl-β-D-glucosaminide (Sigma, cat. no. N9376) to a concentration of 15 mM. Divide into 2-ml aliquots and store up to 1 year at −20°C.
Histodenz stock solution, 88.38% (w/v)
Add 44.19 g Histodenz (Sigma, cat. no. D2158) to a 50-ml tube—the powder will have a volume of approximately 50 ml. Add a small amount of Milli-Q water, cap the tube, and invert until the water is absorbed into the powder. The volume of the powder will decrease as it goes into solution. Add more water up to a volume of 50 ml and repeat a few times until the volume stays at ∼50 ml. Store up to 1 month in a light-proof box at 4°C.
Laemmli lysis buffer
To a 50-ml tube, add 1 g sodium dodecyl sulfate (SDS; Fisher, cat. no. 151-21-3), 1.65 ml of 1 M Tris·Cl (Promega, cat. no. H5121) stock, pH 6.8, 2.5 ml glycerol (Fisher, cat. no. 56-81-5), and Milli-Q water up to 50 ml. Place tube on a plate rocker and allow to mix until solution is clear. Store at room temperature for up to 1 month. Do not refrigerate or SDS will precipitate.
MAPT uptake assay buffer, 1.33×
- 1.5 M sucrose (Fisher, cat. no. BP220) stock (see recipe)
- 100 mM MOPS (Sigma, cat. no. M1254) stock, pH 7.2 (see recipe)
- 100 mM ATP (Sigma, cat. no. A2383) stock
- Recombinant HSPA8 (AbCam, cat. no. 78431)
- MAPT/Tau (Sino Biological, cat. no. 10058-H07E)
- Combine each component in Milli-Q water to final concentrations of 300 mM sucrose, 10 mM MOPS, 10 mM ATP, 0.01 μg/μl HSPA8, and 0.01 μg/μl MAPT. Make fresh before each use. Keep on ice.
MOPS stock solution, 100 mM, pH 7.2
Place 1.05 g MOPS (Sigma, cat. no. M1254) in a 50-ml conical tube. Add Milli-Q water to the 40-ml mark. Cap the tube and shake vigorously until the powder is dissolved. Using a pH meter, adjust the pH to 7.2-7.3 using 0.1 M HCl or 0.1 M NaOH. Add Milli-Q water to 50 ml. Store up to 1 month at 4°C.
MOPS/sucrose buffer (10 mM MOPS, pH 7.2, 300 mM sucrose)
To a 15-ml conical tube, add 1 ml of 100 mM MOPS stock, pH 7.2 (see recipe), 2 ml of 1.5 M sucrose stock (see recipe), and 7 ml Milli-Q water. Cap and invert to mix. Make fresh for each experiment. Store no more than 1 day at 4°C.
Sucrose stock solution, pH 7.2-7.3, 0.25 M
Place 4.28 g sucrose (Fisher, cat. no. BP220) in a 50-ml conical tube. Add Milli-Q water to the 40-ml mark. Cap the tube and rock it on a plate rocker until all of the sucrose is dissolved. Using a pH meter, adjust the pH to 7.2-7.3 using 0.1 M HCl or 0.1 M NaOH. Add Milli-Q water to 50 ml. Store up to 1 month at 4°C.
Sucrose stock solution, 1.5 M
Add 25.67 g sucrose (Fisher, cat. no. BP220) to a 50-ml conical tube. Add Milli-Q water to the 50-ml mark and rock the tube on a plate rocker until all of the sucrose dissolves. Store up to 1 month at 4°C.
COMMENTARY
Background Information
Isolation of lysosome-enriched fractions has been essential for understanding the basic biology of CMA. However, many of the foundational discoveries in the CMA field relied on the use of metrizamide as a separation medium for the preparation of lysosome fractions. Because metrizamide is now extraordinarily expensive and difficult to obtain, it is necessary to use an affordable alternative capable of producing similar results. The protocols described here explain how to use Histodenz as a replacement for metrizamide, allowing researchers to repeat and expand upon the foundational CMA studies.
Critical Parameters
Effects of the circadian cycle and nutritional state on autophagy
The relative activity of both macroautophagy and CMA oscillates with the circadian cycle (Di Ma et al., 2012; Juste et al., 2021). In C57BL/6J mice maintained on a 12 hr light/12 hr dark cycle, CMA activity peaks near the beginning of the light phase (Juste et al., 2021). Therefore, most CMA studies will begin lysosome isolation at the beginning of the day. It is critically important that if multiple experimental replicates are being performed on different days, the dissections are performed at the same time each day.
Moreover, the activity of macroautophagy and CMA is increased by fasting (Cuervo et al., 1995). In most cases, it will be appropriate to fast the mice for 18 hr before collecting liver tissue for lysosome isolation. However, there are several exceptions. If the goal of the lysosome isolation is to investigate the actions of genetic alterations or drug treatments that enhance baseline CMA activity (in fed animals), then it will be appropriate to use ad libitum –fed animals. For example, long-lived pou1f1 mutant (Snell dwarf) mice and ghr KO mice have elevated CMA, even when they are fed ad libitum (Endicott, Boynton et al., 2020; Endicott et al., 2021). Mice treated with inhibitors of class I PI3K, such as buparlisib, also have enhanced CMA when fed (Endicott, Ziemba et al., 2020). Individual investigators should carefully plan their experimental objectives to decide whether fasting the mice before lysosome isolation is appropriate.
Sample numbers, batch effects, and sex as a biological variable
When using this protocol, the number of samples that can be prepared in one batch is limited to a number that will safely balance the swinging-bucket rotor of an ultracentrifuge (i.e., 2, 3, 4, or 6). Experimental groups should be laid out in a way that can be accommodated by this assay. Experiments should be laid out such that all experimental groups are equally represented in each batch.
There is extensive literature showing organ-specific sexual dimorphisms in macroautophagy and lysosomal gene expression in mice and rats (Shang et al., 2021). Sex differences in macroautophagy are especially relevant to sex differences in the progression of cancer, heart disease, and neurodegeneration (Du et al., 2009; Koenig et al., 2014; Shang et al., 2021). Despite the known effects of biological sex on macroautophagy, effects of sex on CMA are poorly studied in mammals. Knockout of the lamp2a gene in medaka (Japanese rice fish) leads to a female-specific dysregulation of carbohydrate metabolism in the liver (Lescat et al., 2020), similar to what is observed in mice with a liver-specific lamp2a deletion (Schneider et al., 2014). We have recently reported that there are sex-specific differences in the expression of LAMP2A in the brain of genetically heterogenous mice, and that liver lysosomes of male mice have more CMA substrate uptake activity than those from female mice (Zhang, Zhang et al., 2023). Thus, in some cases, users of this protocol might need to account for sex as a biological variable. In such situations, each experimental batch should include both male and female mice. If lysosome-enriched fractions from males and females are prepared in separate batches, then it will not be possible to distinguish if any observed differences arise from genuine biology or from artifacts of slight variations in batch preparation.
Effects of confluence on CMA in cultured cells
We have recently reported that CMA activity in cultured AML12 cells increases with increasing confluence (Zhang, Burns et al., 2023). The mechanism accounting for this increased CMA activity has not been characterized. However, with increasing confluence, there is a significant increase in the expression of the CMA receptor LAMP2A and CMA activator PTEN, and there is a decrease in AKT phosphorylation (Zhang, Burns et al., 2023). Decreasing AKT phosphorylation activates CMA (Arias et al., 2015; Endicott, Ziemba et al., 2020). In studies of CMA in cultured cells, it is critical that confluence be carefully monitored and controlled between experimental replicates.
Cell lysis by nitrogen cavitation
Before beginning the Alternate Protocol, it is necessary to evaluate and optimize the lysis conditions for the cultured cells, especially if using a different cell line from AML12, for which this protocol was optimized. The most critical parameter to optimize is the pressure of nitrogen in the cavitation vessel. To perform this optimization, perform steps 3-9 of the Alternate Protocol, and then load the cell suspension into the cavitation vessel and seal the lid. Hook the apparatus to the compressed nitrogen tank, and add 100 psi. Perform steps 11 and 12 (which describes how to check for cell lysis). If there is insufficient cell breakage, load the cell suspension back into the cavitation vessel and increase the pressure in 100-psi steps until sufficient cell lysis is achieved.
Yield and scale for lysosome-enriched fractions from cultured cells
The Alternate Protocol yields ∼100 μg of lysosomal protein from four T175 flasks of AML12 cells at 90% confluence. As we have recently described (Zhang, Burns et al., 2023), the expression of lysosomal proteins increases with confluence in AML12 cells. Thus, in conditions where it is important to produce a yield of >100 μg of lysosomal protein, cells should be grown to 100% confluence and additional flasks of cells should be used.
Troubleshooting
A list of potential problems and suggested solutions are presented in Table 1.
Problem | Possible cause | Solution |
---|---|---|
Bands on gradient look diffuse | Too much mixing between layers | Practice preparing gradients with layers dyed with food coloring, until no mixing between layers is observed |
No bands on gradient | Gradient was tilted or shaken before or after ultracentrifugation | Take care not to tilt gradients |
High percentage of broken lysosomes | Vortexing, aggressive pipetting during resuspension | Pipet lysosomes slowly and gently |
No uptake of CMA substrates | Protease inhibitor was expired or too dilute | Use fresh, concentrated protease inhibitors |
CMA substrate is not appropriate | Use the product listed in this protocol as a positive control |
Statistical Analysis
The statistics used to analyze the results of these experiments are straightforward. In most cases, an unpaired t test is sufficient to evaluate for differences between experimental groups. In Figure 1E, we show data from both male and female mice. In this case a “full-model” two-factor ANOVA is appropriate to evaluate whether the sex of the animals affects the distribution of the results.
Understanding Results
Each method presented in this paper includes a brief description of how to analyze data. A point where there is likely to be some confusion is in the calculation of the fold enrichment of lysosomal-resident proteins in lysosomal fractions. Because of the very high enrichment of lysosomal proteins attained by these protocols, western blotting requires special considerations. It is necessary to load 10-fold less protein from the lysosome fraction into protein gels than from the whole-liver (or whole-cell) lysates, in order to be able to detect bands from both of these fractions in the linear range of the imaging system. When calculating relative enrichment of lysosomal proteins from the western data, it is therefore important to remember to multiply the band intensity (and standard errors) from the lysosome fractions by a factor of 10.
Time Considerations
Because Support Protocol 2 and Support Protocol 3 must be performed on the same day as the Basic Protocol, it is critical to carefully manage the time it takes to complete each experiment. Downtime must be used to set up the next experimental step. Table 2 provides an estimation of the amount of time necessary to complete each step.
Basic Protocol | ||
---|---|---|
Step(s) | Description | Time (min) |
Basic Protocol | ||
1 | Setup | 15 |
2-5 | Mouse dissections | 45 |
6-14 | Liver lysis | 30 |
15-16 | Gradient preparation | 20 |
17-18 | Ultracentrifuge setup | 15 |
19 | Ultracentrifuge spin | 120 |
20 | Ultracentrifuge disassembly | 15 |
21-22 | Fraction collection | 15 |
23 | Centrifugation | 30 |
24 | Wash | 20 |
25 | Resuspension | 15 |
26 | Protein quantification | 45 |
27 | Volume adjustments | 10 |
Total: | 6 hr and 35 min | |
Support Protocol Support Protocol 2a | ||
1-3 | Set up | 30 |
4 | Add lysosomes | 10 |
5 | Incubation | 20 |
6 | Centrifugation | 15 |
7-8 | Buffer change | 15 |
9 | Incubation | 10 |
10 | Centrifugation | 15 |
11 | Remove supernatant | 10 |
Total: | 2 hr and 5 min |
- a Perform Support Protocol 3, steps 1-9, in parallel with Support Protocol 2.
Acknowledgments
Mouse husbandry at the University of Michigan was conducted by Lori Roberts, Ilkim Erturk, Jacob Sheets, Micah Bush, and Caitlin Reynolds.
We thank the Department of Pathology and the Department of Cell & Developmental Biology for allowing us to use shared resources including ultracentrifuges and rotor assemblies. This work was supported by the Hevolution Foundation grant HF-AGE 005 to Endicott, the NIH grant P30 AG 024824 (Claude D. Pepper Older Adults Independence Center), and the NIH grant U19-AG023122 to Miller.
Author Contributions
Calvin Burns : Investigation; methodology; validation; visualization; writing—original draft; writing—review and editing. Richard Miller : Conceptualization; funding acquisition; project administration; supervision; writing—original draft; writing—review and editing. Joseph Endicott : Conceptualization; funding acquisition; investigation; methodology; project administration; supervision; validation; visualization; writing—original draft; writing—review and editing.
Conflict of Interest
The authors have no conflicts of interest to declare.
Open Research
Data Availability Statement
Data are available upon request from the corresponding author (jendicot@med.umich.edu).
Literature Cited
- Agarraberes, F. A., & Dice, J. F. (2001). A molecular chaperone complex at the lysosomal membrane is required for protein translocation. The Journal of Cell Science , 114, 2491–2499. https://doi.org/10.1242/jcs.114.13.2491
- Aniento, F., Roche, E., Cuervo, A. M., & Knecht, E. (1993). Uptake and degradation of glyceraldehyde-3-phosphate dehydrogenase by rat liver lysosomes. The Journal of Biological Chemistry , 268, 10463–10470. https://doi.org/10.1016/S0021-9258(18)82222-0
- Arias, E., Koga, H., Diaz, A., Mocholi, E., Patel, B., & Cuervo, A. M. (2015). Lysosomal mTORC2/PHLPP1/Akt regulate chaperone-mediated autophagy. Molecular Cell , 59, 270–284. https://doi.org/10.1016/j.molcel.2015.05.030
- Bandyopadhyay, U., Kaushik, S., Varticovski, L., & Cuervo, A. M. (2008). The chaperone-mediated autophagy receptor organizes in dynamic protein complexes at the lysosomal membrane. Molecular Cell , 28, 5747–5763. https://doi.org/10.1128/MCB.02070-07
- Chiang, H.-L., Terlecky, S. R., Plant, C. P., & Dice, J. F. (1989). A role for a 70-kilodalton heat shock protein in lysosomal degradation of intracellular proteins. Science , 246, 382–385. https://doi.org/10.1126/science.2799391
- Cuervo, A. M., & Dice, J. F. (1996). A receptor for the selective uptake and degradation of proteins by lysosomes. Science , 273, 501–503. https://doi.org/10.1126/science.273.5274.501
- Cuervo, A. M., & Dice, J. F. (2000). Age-related decline in chaperone-mediated autophagy. Journal of Biological Chemistry , 275, 31505–31513. https://doi.org/10.1074/jbc.M002102200
- Cuervo, A. M., Dice, J. F., & Knecht, E. (1997). A population of rat liver lysosomes responsible for the selective uptake and degradation of cytosolic proteins. The Journal of Biological Chemistry , 272, 5606–5615. https://doi.org/10.1074/jbc.272.9.5606
- Cuervo, A. M., Knecht, E., Terlecky, S. R., & Dice, J. F. (1995). Activation of a selective pathway of lysosomal proteolysis in rat liver by prolonged starvation. The American Journal of Physiology , 269, C1200–C1208. https://doi.org/10.1152/ajpcell.1995.269.5.C1200
- Cuervo, A. M., Terlecky, S. R., Dice, J. F., & Knecht, E. (1994). Selective binding and uptake of ribonuclease A and glyceraldehyde-3-phosphate dehydrogenase by isolated rat liver lysosomes. Journal of Biological Chemistry , 269, 26374–26380. https://doi.org/10.1016/S0021-9258(18)47204-3
- Dice, J. F. (1990). Peptide sequences that target cytosolic proteins for lysosomal proteolysis. Trends in Biochemical Sciences , 15, 305–309. https://doi.org/10.1016/0968-0004(90)90019-8
- Du, L., Hickey, R. W., Bayir, H., Watkins, S. C., Tyurin, V. A., Guo, F., Kochanek, P. M., Jenkins, L. W., Ren, J., Gibson, G., Chu, C. T., Kagan, V. E., & Clark, R. S. (2009). Starving neurons show sex difference in autophagy. The Journal of Biological Chemistry , 284, 2383–2396. https://doi.org/10.1074/jbc.M804396200
- Endicott, S. J., Boynton, D. N., Beckmann, L. J., & Miller, R. A. (2020). Long-lived mice with reduced growth hormone signaling have a constitutive upregulation of hepatic chaperone-mediated autophagy. Autophagy , 17(3), 612–625. https://doi.org/10.1080/15548627.2020.1725378
- Endicott, S. J., Monovich, A. C., Huang, E. L., Henry, E. I., Boynton, D. N., Beckmann, L. J., MacCoss, M. J., & Miller, R. A. (2021). Lysosomal targetomics of ghr KO mice shows chaperone-mediated autophagy degrades nucleocytosolic acetyl-CoA enzymes. Autophagy , 18(7), 1551–1571. https://doi.org/10.1080/15548627.2021.1990670
- Endicott, S. J., Ziemba, Z. J., Beckmann, L. J., Boynton, D. N. Jr., & Miller, R. A. (2020). Inhibition of class I PI3K enhances chaperone-mediated autophagy. The Journal of Cell Biology , 219(12), e202001031. https://doi.org/10.1083/jcb.202001031
- Hao, Y., Kacal, M., Ouchida, A. T., Zhang, B., Norberg, E., & Vakifahmetoglu-Norberg, H. (2019). Targetome analysis of chaperone-mediated autophagy in cancer cells. Autophagy , 15, 1558–1571. https://doi.org/10.1080/15548627.2019.1586255
- Johansen, T., & Lamark, T. (2014). Selective autophagy mediated by autophagic adapter proteins. Autophagy , 7, 279–296. https://doi.org/10.4161/auto.7.3.14487
- Juste, Y. R., Kaushik, S., Bourdenx, M., Aflakpui, R., Bandyopadhyay, S., Garcia, F., Diaz, A., Lindenau, K., Tu, V., Krause, G. J., Jafari, M., Singh, R., Muñoz, J., Macian, F., & Cuervo, A. M. (2021). Reciprocal regulation of chaperone-mediated autophagy and the circadian clock. Nature Cell Biology , 23, 1255–1270. https://doi.org/10.1038/s41556-021-00800-z
- Kacal, M., Zhang, B., Hao, Y., Norberg, E., & Vakifahmetoglu-Norberg, H. (2021). Quantitative proteomic analysis of temporal lysosomal proteome and the impact of the KFERQ-like motif and LAMP2A in lysosomal targeting. Autophagy , 17(11), 3865–3874. https://doi.org/10.1080/15548627.2021.1876343
- Koenig, A., Sateriale, A., Budd, R. C., Huber, S. A., & Buskiewicz, I. A. (2014). The role of sex differences in autophagy in the heart during coxsackievirus B3-induced myocarditis. Journal of Cardiovascular Translational Research , 7(2), 182–191. https://doi.org/10.1007/s12265-013-9525-5
- Lescat, L., Véron, V., Mourot, B., Peron, S., Chenais, N., Dias, K., Riera-Heredia, N., Beaumatin, F., Pinel, K., Priault, M., Panserat, S., Salin, B., Guiguen, Y., Bobe, J., Herpin, A., & Seiliez, I. (2020). Chaperone-mediated autophagy in the light of evolution: Insight from fish. Molecular Biology and Evolution , 37, 2887–2899. https://doi.org/10.1093/molbev/msaa127
- Ma, D., Li, S., Molusky, M. M., & Lin, J. D. (2012). Circadian autophagy rhythm: A link between clock and metabolism? Trends in Endocrinology & Metabolism, 23, 319–325.
- Mizushima, N., & Levine, B. (2010). Autophagy in mammalian development and differentiation. Nature Cell Biology , 12(9), 823–830. https://doi.org/10.1038/ncb0910-823
- Murrow, L., & Debnath, J. (2013). Autophagy as a stress-response and quality-control mechanism: Implications for cell injury and human disease. Annual Review of Pathology: Mechanisms of Disease , 8, 105–137. https://doi.org/10.1146/annurev-pathol-020712-163918
- Ryter, S. W., Cloonan, S. M., & Choi, A. M. K. (2013). Autophagy: A critical regulator of cellular metabolism and homeostasis. Molecules and Cells , 36, 7–16. https://doi.org/10.1007/s10059-013-0140-8
- Sahu, R., Kaushik, S., Clement, C. C., Cannizzo, E. S., Scharf, B., Follenzi, A., Potolicchio, I., Nieves, E., Cuervo, A. M., & Santambrogio, L. (2011). Microautophagy of cytosolic proteins by late endosomes. Developmental Cell , 20, 131–139. https://doi.org/10.1016/j.devcel.2010.12.003
- Salvador, N., Aguado, C., Horst, M., & Knecht, E. (2000). Import of a cytosolic protein into lysosomes by chaperone-mediated autophagy depends on its folding state. The Journal of Biological Chemistry , 275, 27447–27456. https://doi.org/10.1016/S0021-9258(19)61529-2
- Schneider, J. L., Suh, Y., & Cuervo, A. M. (2014). Deficient chaperone-mediated autophagy in liver leads to metabolic dysregulation. Cell Metabolism , 20, 417–432. https://doi.org/10.1016/j.cmet.2014.06.009
- Shang, D., Wang, L., Klionsky, D. J., Cheng, H., & Zhou, R. (2021). Sex differences in autophagy-mediated diseases: Toward precision medicine. Autophagy , 17, 1065–1076. https://doi.org/10.1080/15548627.2020.1752511
- Storrie, B., & Madden, E. (1990). Isolation of subcellular organelles. Methods in Enzymology , 182, 203–225. https://doi.org/10.1016/0076-6879(90)82018-W
- Zhang, K. K., Burns, C. M., Skinner, M. E., Lombard, D. B., Miller, R. A., & Endicott, S. J. (2023). PTEN is both an activator and a substrate of chaperone-mediated autophagy. The Journal of Cell Biology , 222(9), e202208150. https://doi.org/10.1083/jcb.202208150
- Zhang, K. K., Zhang, P., Kodur, A., Erturk, I., Burns, C. M., Kenyon, C., Miller, R. A., & Endicott, S. J. (2023). LAMP2A, and other chaperone-mediated autophagy related proteins, do not decline with age in genetically heterogeneous UM-HET3 mice. Aging , 15(11), 4685–4698.