Design and Derivation of Multi-Reporter Pluripotent Stem Cell Lines via CRISPR/Cas9n-Mediated Homology-Directed Repair
Rabea Dettmer, Rabea Dettmer, Ortwin Naujok, Ortwin Naujok
Abstract
During the past decade, RNA-guided Cas9 nuclease from microbial clustered regularly interspaced short palindromic repeats (CRISPR/Cas9) has become a powerful tool for gene editing of human pluripotent stem cells (PSCs). Using paired CRISPR/Cas9 nickases (CRISPR/Cas9n) it is furthermore possible to reduce off-target effects that may typically occur with traditional CRISPR/Cas9 systems while maintaining high on-target efficiencies. With this technology and a well-designed homology-directed repair vector (HDR), we are now able to integrate transgenes into specific gene loci of PSCs in an allele conserving way. In this protocol we describe CRISPR/Cas9n design and homology directed repair vector design, transfection of human pluripotent stem cells and selection and expansion of generated cell clones. © 2020 The Authors.
Basic Protocol 1 : Repair template design and CRISPR/Cas9n construction
Basic Protocol 2 : Transfection of human pluripotent stem cells by electroporation
Basic Protocol 3 : Genotyping of generated cell clones
INTRODUCTION
Pluripotent stem cells (PSCs), such as embryonic stem cells (ESCs) and induced pluripotent stem cells (iPSCs), have a vast differentiation capability. This can be exploited by using them as cell sources for cell replacement therapy and as powerful cellular models to study disease onset and progression in a dish. Both areas require PSCs to be differentiated into stem cell-derived somatic cell types, which is, however, often challenging because of the heterogeneity of the generated cells (Veres et al., 2019).
Using CRISPR/Cas9 technology and homology-directed repair (HDR), transgenes can be integrated into the gene loci of developmental marker genes (Doudna & Charpentier, 2014). Consequently, cells with reporter gene expression can be selected or sorted to improve differentiation efficiencies. To prevent off-target effects coming with the regular CRISPR/Cas9 system, we use in our protocol paired nickases. The CRISPR/Cas9n is mutated either affecting the RuVC domain (D10A mutation) or the HNH domain (H840A). The D10A mutant cleaves only the target strand, whereas the H840A mutant cleaves only the non-target strand (Mali et al., 2013). Therefore, a pair of CRISPR/Cas9n with two guide RNAs (gRNAs) is necessary to create a staggered cut at a genomic site. Particularly for HDR, it is recommended to use the D10A mutant for higher editing efficiency (Bothmer et al., 2017).
With our particular HDR vector design, we conserve the gene function of target genes on both alleles. The transgenes do not displace the target genes, but are expressed along with the host gene under the control of the endogenous promoter (Fig. 1). The transgenes are fused in frame to the host gene in a 3′ prime fashion before the genes’ stop codon. Furthermore, we use self-cleaving peptides (2A-sites) (Szymczak et al., 2004; Torres et al., 2010) in our HDR vector design to separate endogenous proteins from transgene proteins so that all of them stay as unrestricted as possible. With these 2A-sites, it is also possible to generate multi-reporter stem cell lines comprising several different reporter genes in one target gene locus. Therefore, a combination of, e.g., fluorescent reporters, cell surface reporters, or resistance genes is feasible enabling several applications.
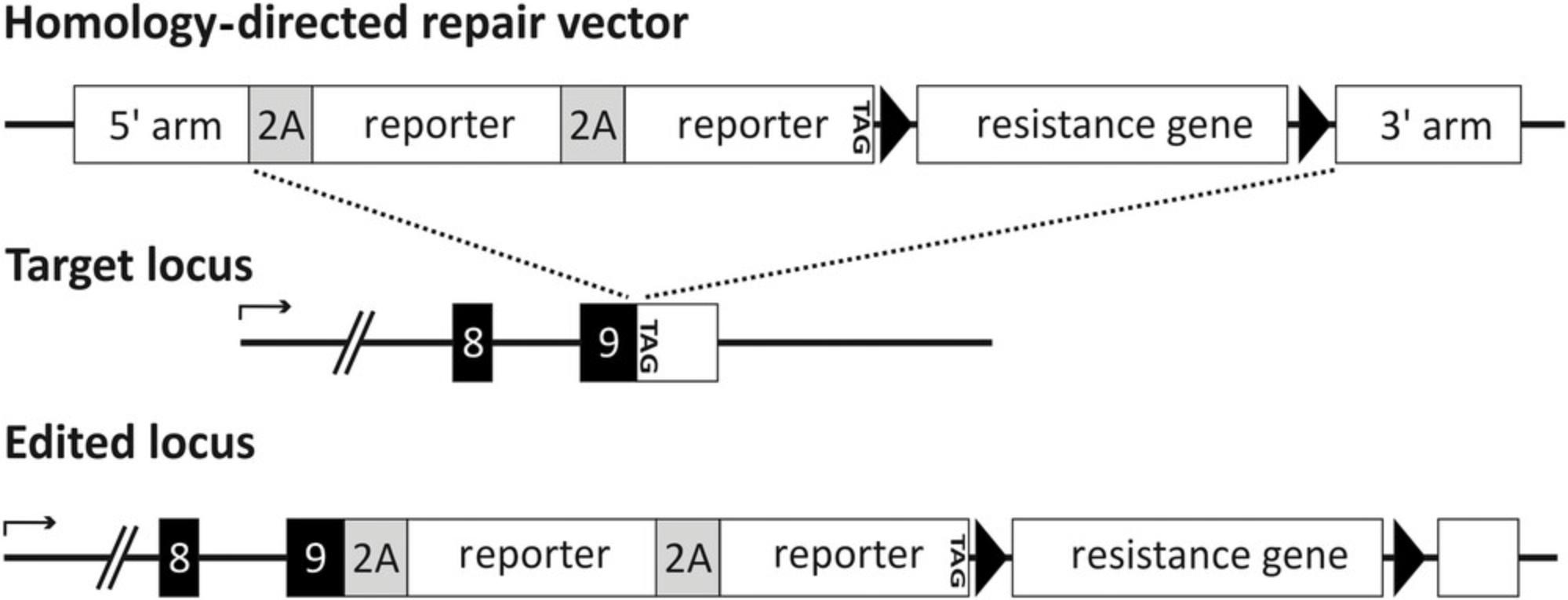
The successful integration of transgenes, e.g., reporter genes, in PSCs enables direct labeling and purification of cell populations at certain stages of differentiation. Consequently, progenitor populations and terminally matured cell types can be purified, downstream differentiation can be improved, and cells of unwanted lineages can be removed. Those transgenic PSCs facilitate the characterization of particular differentiated cell populations in vitro and can give information about developmental processes during human in vitro organogenesis.
In this article, we describe a detailed and stepwise protocol of the generation of pluripotent reporter stem cell lines mediated by the CRISPR/Cas9n system. We describe the design of the repair vector and of CRISPR/Cas9n (Basic Protocol 1), the transfection of stem cells via electroporation (Basic Protocol 2), and genotyping of generated cell clones (Basic Protocol 3).
Basic Protocol 1: REPAIR TEMPLATE DESIGN AND CRISPR/CAS9n CONSTRUCTION
In order to precisely modify a genome at a desired target region, homologous recombination can be used. Here plasmid-based donor repair templates with homology arms are utilized. Homology arms are usually defined as genomic fragments flanking the transgene. The length of the homology arms can vary, but should include around 500 bp, if the modification is large and comprises reporter genes like fluorescent proteins. Most targeting vectors are typically used to replace a part of the coding sequence of an endogenous gene or all of it. This leads to loss-of-function mutations on at least one allele. With the use of 2A-sites, it is possible to preserve the gene function on both alleles of the modified cells and additionally integrate and co-express multiple reporter genes in one gene locus. For this purpose, reporter genes need to be placed in frame with the endogenous genetic code prior to the endogenous stop-codon (Fig. 1). The endogenous gene and the reporter genes ought to be separated with 2A-sites. With the addition of a 2A-site, 15-20 amino acids are added to the C-terminus of the prior gene. There are several 2A-sites available, the four most widely used are P2A (derived from porcine teschovirus-1), F2A (derived from foot-and-mouth disease virus), E2A (derived from equine rhinitis A virus) and T2A (derived from Thoseaasigna virus). The cleavage efficiencies of the different 2A-sites vary according to their position and combination in the construct (Liu et al., 2017); hence, the choice of the 2A-site needs to be considered for each repair vector individually. In addition, a positive selection gene present on the repair vector is needed for the selection of cells that have integrated the repair template via homologous recombination. This positive selection gene must not be driven by the endogenous promoter and needs to precede its own promoter. Since widely used viral promoters such as the cytomegalovirus (CMV), can be efficiently silenced in pluripotent stem cells (Wolf & Goff, 2007), the choice of the promoter is of particular importance. Therefore, tissue-specific promoters or tetracycline-regulated promoters are the promoters of choice (Hartman et al., 2014; Vieyra & Goodell, 2007). The positive selection gene and its promoter are supposed to be floxed to enable their depletion via the Cre-lox system (Missirlis, Smailus, & Holt, 2006; Sternberg, Hamilton, & Hoess, 1981). Restriction sites can be added flanking each genetic element of the repair vector to permit the replacement of the resistance gene and/or the reporter genes. Restriction sites flanking the whole construct excluding the homology arms gives the feasibility to easily transfer constructs for the use in other gene loci.
In our protocol, we use the CRISPR-Cas system derived from Streptococcus pyogenes (SPCas9). Crucial for the Cas9 nuclease accuracy is a 20-nt guide sequence within the gRNA. The guide sequence in the target DNA locus must precede a PAM-sequence (NGG in this system), but the PAM-sequence is not a part of the 20-nt guide sequence within the gRNA. The coordinate function of two catalytic domains, RuvC and HNH, of Cas9 nuclease are responsible for its cleavage activity. RuvC cleaves the non-target strand whereas HNH cleaves the target strand. Together these domains generate blunt-ended double-strand breaks (Cong et al., 2013). The D10A mutant Cas9 nickase (Cas9n) produces a single DNA strand cleavage (a nick) on the target strand. Only the combination of Cas9n and two gRNAs targeting opposite DNA strands in a defined proximity leads to a staggered double-strand break (DSB) with overhangs. Cas9n nicks the DNA at ∼3 bp upstream of the PAM-sequence. For high-editing efficiency, the gRNAs should be orientated in PAM-out orientation (Ran et al., 2013) and the nick-sites should have a distance of 37-68 nt (Fig. 2). The DSB should occur approximately 8-20 bp around the stop-codon of the target gene to integrate your transgenes so that the transgenes can be expressed along with the endogenous target gene. To ease the process of finding suitable target sequences, it is beneficial to use online tools such as CCTOP (https://cctop.cos.uni-heidelberg.de) or others for calculation. If you are not able to find suitable gRNA-pairs, the non-mutated Cas9 with only one gRNA for DSB may be used.
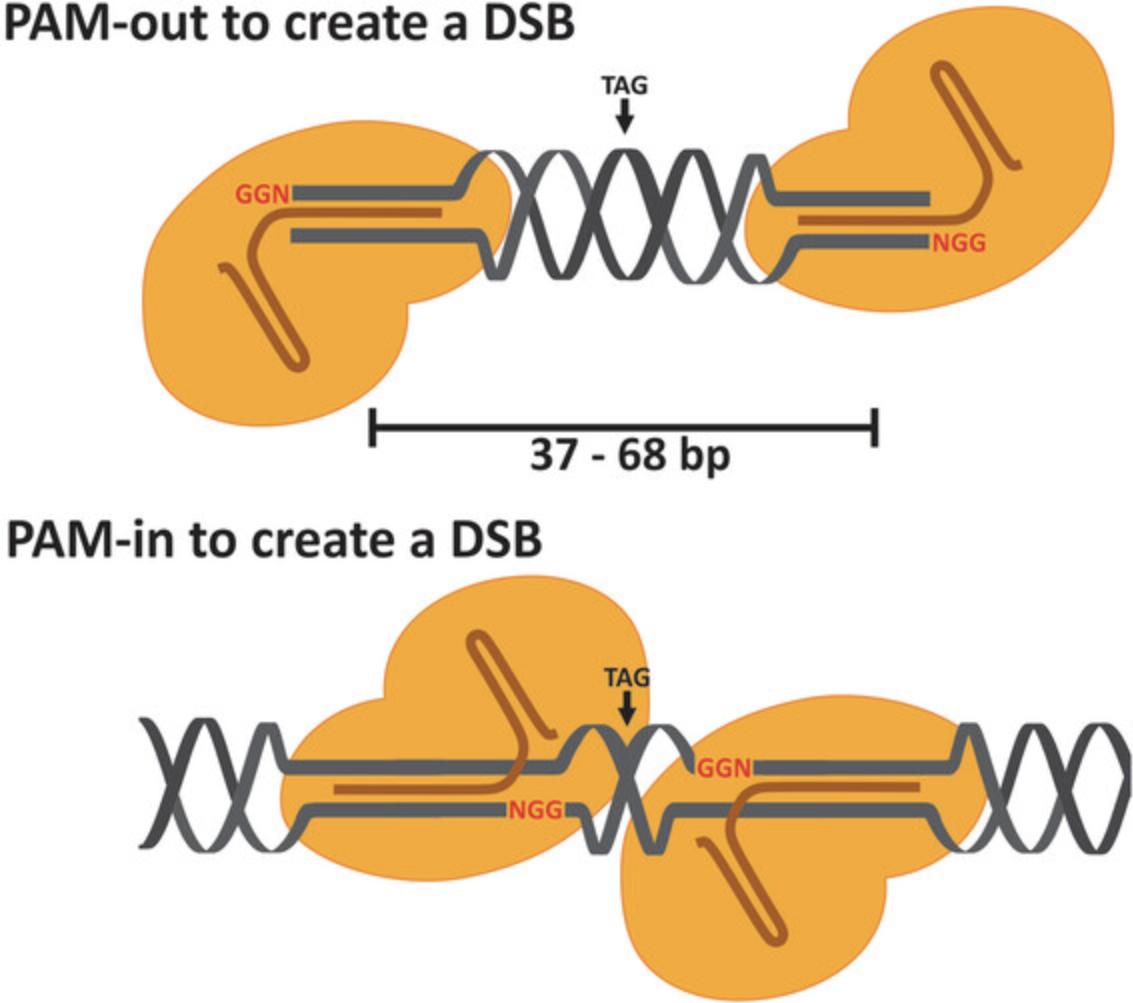
Materials
-
Plasmid: pX335-U6-Chimeric_BB-CBh-hSpCas9n (Addgene plasmid #42335) or other CRISPR/Cas9n (D10A) plasmid of your choice
-
Oligonucleotides for your gRNA (50 nmol), and U6-forward primer ordered at genomic scale (50 nmol)
-
T4 Polynucleotide Kinase (10 U/µl) (Thermo Scientific™, cat. no. EK0031)
-
ATP Solution (10 mM) (Thermo Scientific™, cat. no. PV3227)
-
Bbs I (NEB, cat. no. R0539L)
-
NEBuffer™ 3 (NEB, cat. no. B7003S)
-
FastAP Thermosensitive Alkaline Phosphatase (1 U/µl) (Thermo Scientific™, cat. no. EF0654)
-
TBE Buffer (Tris-borate-EDTA; 10×) (Thermo Scientific™, cat. no. B52)
-
UltraPure™ Agarose (Invitrogen™, cat. no. 16500100)
-
DNA stain (e.g., Midori Green Advance, Nippon Genetics, cat. no. MG04)
-
DNA loading dye (e.g., Orange DNA Loading Dye (6×), Thermo Scientific™, cat. no. R0631)
-
DNA-extraction kit (NucleoSpin Gel and PCR Clean‑up, Macherey-Nagel, cat. no. 740609.50)
-
T4-DNA Ligase and Buffer (Invitrogen™, cat. no. 15224041)
-
Competent E. coli (e.g., One Shot™ TOP10 Chemically Competent E. coli , Thermo Scientific™, cat. no. C404003)
-
LB agar plates (e.g., LB-Agar, Invitrogen™, cat. no. 22700025) supplemented with 50 µg/ml ampicillin (e.g., Sigma-Aldrich, cat. no. A9393)
-
LB medium (e.g., LB Broth Base, Invitrogen™, cat. no. 12780052) supplemented with 50 µg/ml ampicillin
-
Plasmid purification kit (e.g., NucleoBond Xtra Maxi Columns for transfection-grade plasmid DNA, Macherey-Nagel, cat. no. 740414.10S)
-
PCR thermal cycler
-
Microbiological incubator
-
Erlenmeyer flasks
-
Electrophoresis equipment
-
UV table
1.Order your CRISPR/Cas9n plasmid and a pair of oligonucleotides for each gRNA with the overhangs CACC for the sense primer and AAAC for the antisense primer. Note that the gRNA needs to start with a G. If that is not the case, add a 3′ G to the CACC sequence.
For example:
- CACC G NNNNNNNNNNNNNNNNN
- AAAC NNNNNNNNNNNNNNNNNC
2.Phosphorylate your oligos as follows:
0.5 μl | Sense primer (100 μm) |
0.5 μl | Antisense primer (100 μm) |
1 μl | T4 PNK buffer A |
05 μl | T4 PNK |
1 μl | ATP (10 mM) |
6.5 μl | H2O |
10 μl | total. |
Use the following PCR program:
20 min | 37°C |
10 min | 75°C |
Pause | Cool down to 4°C |
Digest your plasmid
3.For pX335-U6-Chimeric_BB-CBh-hSpCas9n use the following:
X μl | 1 μg vector |
2 μl | NEB buffer 3 |
1 μl | BbsI |
X μl | H2O |
20 μl | Total. |
Incubate at 37°C in a thermal cycler for at least 1 hr or alternatively overnight (with less enzyme). Then add 1 µl of FastAP to remove phosphate groups from the overhangs and incubate for an additional 15-20 min.
4.Gel-purify the vector as follows:
-
For a 1% agarose gel, mix 25 ml TBE Buffer with 250 mg agarose in an Erlenmeyer flask.
-
Warm the mixture, e.g., in the microwave oven until all of the agarose is solved, avoid boil over.
-
Add a DNA visualizing reagent to the liquid gel according to the manufacturer's instructions (e.g., Midori Green Advance) and pour it into a suitable gel chamber provided with a suitable gel comb.
-
Let the gel polymerize for about 20-30 min.
-
Transfer the gel into the electrophoresis chamber filled with TBE Buffer.
-
Load the PCR products with loading dye to the agarose gel and start the electrophoresis (100 mV, ∼30 min).
-
After electrophoresis, visualize the DNA bands using a UV table and cut out the digested vector. Use a gel extraction kit to purify the vector according to the manufacturer's instructions.
5.Ligate your phosphorylated oligomers with your CRISPR/Cas9n vector. Combine the following:
X μl | ∼30-50 ng purified CRISPR/Cas9n vector (step 4) |
1 μl | Annealed and phosphorylated oligo duplex (1:250) (step 2) |
1 μl | T4 DNA ligase buffer |
1 μl | T4 DNA ligase |
X μl | H2O |
10 μl | total. |
Set up a second ligation without insert control (re-ligation control). Incubate according to the manufacturer's instructions provided with the T4-DNA ligase and buffer (Invitrogen). In general, 60-120 min at room temperature gives good results.
6.Transform the ligation into chemical competent or electrocompetent E. coli according to the manufacturer's instructions.
7.Pick colonies and transfer them to ca. 3 ml LB medium each, containing 50 µg/ml ampicillin.
8.Incubate for 8-12 hr on a shaker at 260 rpm and 37°C and then perform a Miniprep with a custom plasmid purification kit. Keep 0.5-1 ml of each E. coli culture and store it up to 2 weeks at 4°C for potential Midi-or Maxipreps after sequencing.
9.Send the plasmid for sequencing using the U6-fwd primer (GAGGGCCTATTTCCCATGATTCC).
10.Prepare a Midi-or Maxiprep of the CRISPR/Cas9n clones with the correct sequence for further use in Basic Protocol 2.
Basic Protocol 2: TRANSFECTION OF HUMAN PLURIPOTENT STEM CELLS BY ELECTROPORATION
Transfections are used to introduce plasmid DNA into cells. In this protocol, we use electroporation, which yields high amounts of transient transfected cells by high-voltage shocks that increase the permeability of cell membranes. The procedure is relatively simple and quick to perform. Cells are converted into single-cell suspension in an electroporation buffer and plasmid DNA is added. The cell-DNA-mixture is transferred to an electroporation cuvette and then exposed to a high-voltage electrical pulse. The magnitude and length of the electrical pulse needs to be adjusted to every cell line particularly to optimize transfection rate and avoid high amounts of cell death. This optimization can be done by optimization protocols, which can be found in the manufacturer's instructions of your electroporation system. Successfully transfected cells need to be cultured under selection pressure. Therefore, it is necessary to determine the optimal concentration of the antibiotic whose resistance gene was transmitted along with the transgenes by transfection. The concentration should be as low as possible and as high as needed to selectively kill non-resistant cells. The antibiotic concentration should lead to cell death of all non-edited cells within 1 week. After transfection, clonal cell lines can be expanded under selective pressure and genotyped (Fig. 3).
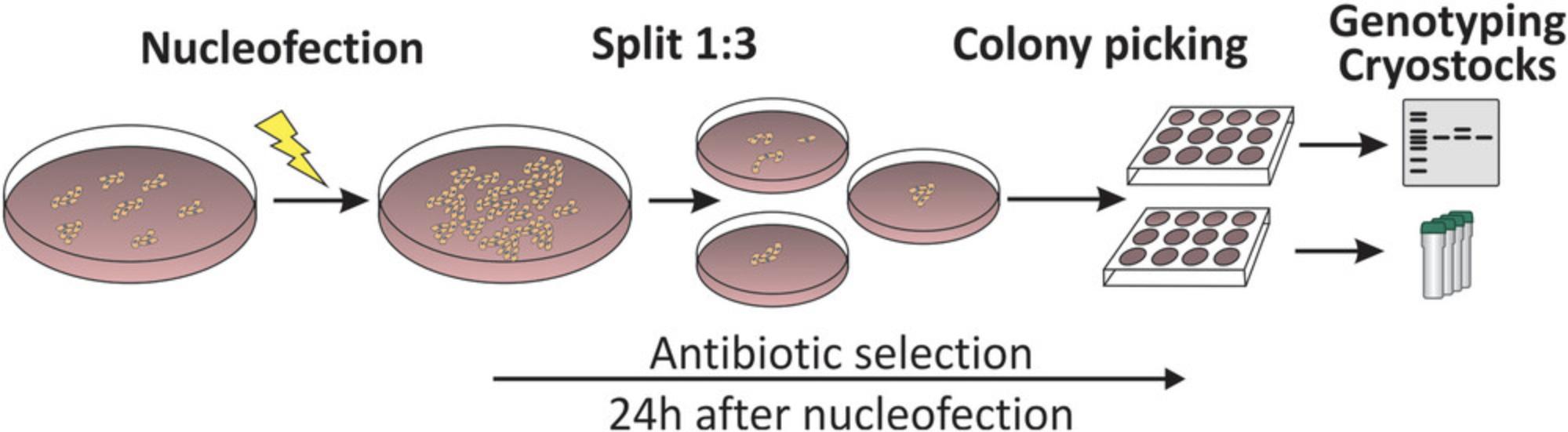
Materials
-
Human pluripotent stem cells (PSCs)
-
PBS w/o Ca2+/Mg2+ (PBS; Sigma-Aldrich, cat. no. D1408)
-
0.25% Trypsin-EDTA solution (T/E) (e.g., Sigma-Aldrich, cat. no. T3924)
-
DMEM/F-12 (Life Technologies, Darmstadt, Germany)
-
Fetal bovine serum (FBS; e.g., Sigma-Aldrich, cat. no. F2442)
-
mTeSR™1 culture medium (STEMCELL Technologies, cat. no. 05850)
-
ROCK inhibitor, Y-27632 (Selleck Chemicals, cat. no. S1049)
-
Matrigel hESC-qualified matrix (Corning, cat. no. 354277)
-
Antibiotics (e.g., Blasticidin)
-
Transfection kit for electroporation of cells (Neon™ Transfection System 100 μl-Kit, Thermo Fisher Scientific, cat. no. MPK10025)
-
CRISPR/Cas9n Plasmids and repair template
-
Non-enzymatic passaging solution such as ReleaSR™ (STEMCELL Technologies, cat. no. 05872)
-
Collagenase type IV (1 mg/ml) (STEMCELL Technologies, cat. no. 07909)
-
Cell culture plastic plates, dishes or flasks including:
- 6-well (Corning/Falcon cat. no. 353046), 12-well, 24-well, and 96-well plates
- 1.5-ml reaction vials
- 100-μl tips
-
37°C, 5% CO2 incubator
-
Centrifuge
-
Cell counting device
-
Light microscope
-
Electroporation device for cells (Neon™ Transfection System, Thermo Fisher scientific, cat no. MPK5000 or Nucleofector™, Lonza, cat. no. AAB-1001)
Optimization of transfection conditions
1.Run an optimization protocol with your chosen PSC line. Optimization protocols can be found in the manufacturer's instructions of your electroporation system.
Determination of sufficient antibiotic concentration for clonal selection
Determine the optimal concentration of the antibiotic you planned to use for selection of successful transfected cells. Be sure that the concentration is sufficient by using concentration series.
2.Wash one or two 80% confluent grown PSC culture cavities of a 6-well plate once with 500 µl PBS.
3.Add 500 µl T/E (trypsin/EDTA) and incubate 4-7 min at 37°C.
4.Add 1 ml DMEM-F12/10% FBS and resuspend the cells a few times.
5.Centrifuge the cell suspension for 5 min at 450 × g , 20-25°C. Resuspend the cell pellet in 1 ml mTeSRTM1 supplemented with 10 µM ROCK inhibitor.
6.Count the cells and seed 100,000 cells per 12-well cavity on a Matrigel-coated 12-well plate in mTeSRTM1 supplemented with 10 µM ROCK inhibitor.
7.The next day change the culture medium to mTeSRTM1 supplemented with the different antibiotic concentrations.
8.Culture the cells for approximately 1 week under visual observation of cell viability and select the lowest concentration of antibiotic with satisfactory cell death. This antibiotic concentration can then be used for selection of transfected cells.
Transfection of pluripotent stem cells (Neon electroporation system)
9.Observe human PSCs on a Matrigel-coated 6-well plate under a light-microscope to monitor cell health and remove spontaneously differentiated areas manually by aspiration.
10.Wash the cells with 500 µl PBS per 6-well cavity.
11.Add 500 µl T/E and incubate 4-5 min at 37°C in the incubator.
12.Stop the enzymatic reaction with 1 ml DMEM-F12/10% FBS and transfer the cells in a 1.5-ml reaction vial. Centrifuge the cells 5 min at 500 × g , 20-25°C.
13.Resuspend the cell pellet in 1 ml PBS and count the cells. For one transfection using 100-µl tips, ∼2 million cells are required.
14.Transfer the cells into a new 1.5-ml reaction vial and centrifuge again for 5 min at 500 × g , 20-25°C.
15.Resuspend the cells in 100 µl electroporation buffer.
16.Add your pair of Cas9n and your HDR vector in a ratio of 1:1:2 and a total of 4 µg DNA.
17.Carefully mix the cells and plasmid DNA by pipetting up and down.
18.Soak up the cell/DNA mixture with the 100 µl transfection tip, avoid air bubbles.
19.Electroporate the cells.
20.Transfer the cells into one 6-well cavity of a 6-well tissue culture plate in mTeSRTM1 containing 10 µM ROCK inhibitor.
21.Perform a daily medium change with mTeSRTM1.
Selection of transfected cell clones
22.After 24-48 hr, start the antibiotic selection by supplementing mTeSRTM1 with antibiotics for selection.
23.At a time point 72-96 hr after transfection, either perform a non-enzymatic split of the cells in a ratio 1:3 on three cavities of a 6-well plate.
-
Wash the cells once with 500-1000 µl PBS.
-
Add 1000 µl ReleaSR™ to the cells and remove it ∼30 s later.
-
Incubate for 4-6 min at 37°C.
-
Add 1000 µl mTeSRTM1 and gently scratch to help the cells detach from the surface. Use cell saver tips.
-
Transfer 333 µl cell suspension into each 6-well cavity and gently rock the plate to ensure consistent spreading.
Or disperse the cells with T/E, count them and dilute them to one cell per 100 µl. Then transfer 100 µl per well on a prepared, Matrigel-coated 96-well plate in mTeSRTM1 supplemented with 10 µM ROCK inhibitor.
24.Cultivate the cells under selection pressure and a daily medium change with mTeSRTM1 containing the adequate concentration of the suitable antibiotic for about 7-14 days.
Picking of clonal colonies
If you choose to perform the non-enzymatic split, pick clones from each 6-well cavity, transfer them into one 24-well cavity per cell clone and expand them. If you choose to do the single cell seed on a 96-well plate, simply expand the cell clones based from the 96-well plate.
25.Prepare a Matrigel-coated 24-well plate with 250 µl culture medium supplemented with 10 µM ROCK inhibitor.
26.Wash the cells once with 1 ml PBS per 6-well cavity. Then add 1 ml Collagenase Type IV (1 mg/ml) and incubate at least for 7 min at 37°C in the incubator. After that add 1 ml mTeSRTM1.
27.Pick the cell clones with a tip of a 100-µl pipet under a light microscope. Remove the cover of the culture dish, center the wanted colony, align the end of the pipet tip above the colony and gently scratch the bottom of the culture plate to detach the colony.
28.Collect the colony in the pipet tip and transfer the clone directly to one cavity of the prepared 24-well plate, or in an intermediate step at first into a 1.5-ml reaction vial provided with 100 µl mTeSRTM1 supplemented with 10 µM ROCK inhibitor.
29.When the cells in the 24-well cavity have grown to 70%-80% confluency, perform a non-enzymatic split (1:2) on two 12-well cavities per clone.
-
Wash the cells once with 500 µl PBS.
-
Add 500 µl ReleaSR™ to the cells and remove it ∼30 s later.
-
Incubate for 4-6 min at 37°C.
-
Add 500 µl mTeSRTM1 and gently scratch to help the cells detach from the surface. Use cell saver tips.
-
Transfer 250 µl cell suspension into each 12-well cavity and gently rock the plate to ensure consistent dispersion.
30.Expand one of two 12-well cavities of each clone and use the cellular material of the other one for genotyping
Basic Protocol 3: GENOTYPING OF GENERATED CELL CLONES
Positive selection markers such as antibiotic-resistance genes can be used to remove non-edited cells. However, antibiotic selection can incorrectly select for cells with multiple aberrant transgene insertions. In order to identify cell clones harboring the transgene insertion in the desired region, it is required to confirm the correct transgene insertion via genotyping. In order to screen the generated cell clones, primer pairs have to be designed. Cell clones need to be tested for correct transgene insertion and also for the wildtype allele. For genotyping the forward primer 1 needs to bind 5′ upstream of the 5′-homology arm of the transgene. The reverse primer 1 needs to bind in the transgene somewhere downstream of the 5′-homology arm. The distance between forward and reverse primer should span 500 to 2000 bp. To test for the wildtype allele, the same forward primer 1 can be used. The reverse primer 2 should bind downstream of the 3′-homology arm. Since the transgene spans several 100 bp between the homology arms, there should be no PCR-product for the wildtype allele due to the size restrictions during the PCR run. PCR-products can then be visualized by an agarose-gel (Fig. 4). PCR-products of transgene insertions can be sent for sequencing. If transgene insertion PCR and wild-type PCR are both positive, the clone is heterozygous. No wildtype PCR product implies for a homozygous clone and only wildtype bands imply for negative clones. It is advisable to test several different primers and/or primer combinations to increase the likelihood of functional primer pairs.
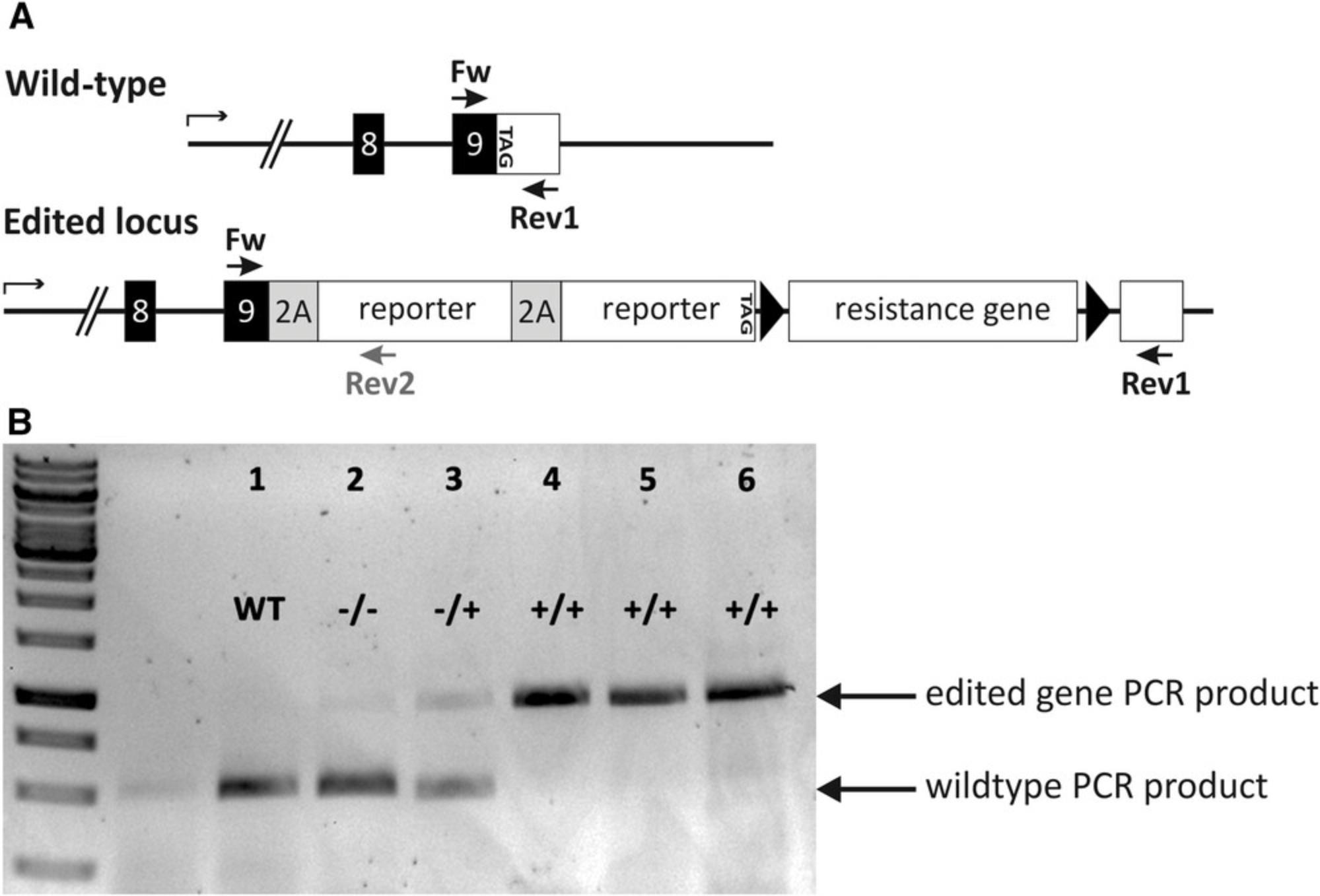
Materials
-
Generated clonal cells (see Basic Protocol 2)
-
Phosphate-buffered saline (PBS) w/o Ca2+/Mg2+ (Sigma-Aldrich, cat. no. D1408)
-
0.25% Trypsin-EDTA solution (T/E; e.g., Sigma-Aldrich, cat. no. T3924)
-
Fetal bovine calf serum (FBS; e.g., Sigma-Aldrich, cat. no. F2442)
-
DMEM/F-12 (Life Technologies)
-
DNA-extraction kit (NucleoSpin Gel and PCR Clean‑up; Macherey-Nagel, cat. no. 740609.50)
-
Previously designed primers for screening
-
DNA Polymerase (Taq DNA Polymerase, BioThermBio™, cat. no. GC-057-0100/Q5® High-Fidelity PCR kit, NEB, cat. no. E0555S)
-
10 mM dNTP Mix (Qiagen, cat. no. 201900)
-
PCR grade water
-
TBE Buffer (Tris-borate-EDTA) (10×) (Thermo Scientific™, cat. no. B52)
-
UltraPure™ Agarose (Invitrogen™, cat. no. 16500100)
-
DNA stain (e.g., Midori Green Advance, Nippon Genetics, cat. no. MG04)
-
DNA loading dye (e.g., Orange DNA Loading Dye (6×), Thermo Scientific™, cat. no. R0631)
-
GeneRuler 1-kb Plus DNA Ladder (Thermo Scientific™, cat. no. SM1331)
-
37°C incubator
-
1.5-ml reaction vials
-
PCR cycler
-
Erlenmeyer flasks
-
Electrophoresis equipment
1.Choose one 12-well cavity per clone for genotyping.
2.Wash the 80%-90% confluent grown cavity with 300 µl PBS.
3.Add 300 µl T/E and incubate for 4-6 min at 37°C in the incubator.
4.Add 700 µl DMEM-F12/10% FBS and resuspend the cells.
5.Transfer the cells in a 1.5 ml reaction vial and centrifuge for 5 min at 500 × g , 20-25°C.
6.Remove the supernatant and keep the cell pellet for DNA-extraction.
7.Extract the genomic DNA using a regular DNA extraction-kit according to the manufacturer's instructions.
8.Set up a PCR-reaction with your extracted genomic DNA and your previously designed primer pairs. Note that it is required to use a polymerase with proofreading function (e.g., Q5® High-Fidelity PCR kit, NEB, cat. no. E0555S) when sequencing of the product is desired. It is also advisable to run a gradient PCR to optimize the annealing temperature.
For standard PCR prepare the following mix :
2.5 μl | 10× PCR Buffer +MgCl2 |
0.5 μl | 10 mM dNTPs |
1 μl | 10 μM forward primer |
1 μl | 10 μM reverse primer |
1 μl | 50 ng/μl genomic DNA |
0.5 μl | 5 U/μl Taq DNA polymerase |
18.5 μl | PCR grade H2O |
25 μl | total. |
For standard PCR, use the following program :
1 cycle: | 5 min | 95°C (initial denaturation) |
40 cycles: | 30 sec | 95°C (denaturation) |
30 sec | 60°C (annealing) | |
1 min 10 sec | 72°C (extension) | |
1 cycle: | 5 min | 72°C (final extension) |
Final step: | Cool down to | 4°C. |
For Q5 PCR conditions prepare the following mix :
5 μl | 5× Q5® Reaction Buffer |
0.5 μl | 10 mM dNTPs |
1.25 μl | 10 μM forward primer |
1.25 μl | 10 μM reverse primer |
2 μl | 50 ng/µl genomic DNA |
0.25 μl | Q5® high-fidelity DNA Polymerase |
5 μl | 5× Q5® high GC-Enhancer (optional) |
9.75 μl | PCR grade H2O |
25μl | total. |
For Q5 PCR conditions, run the following program :
1 cycle: | 30 sec | 98°C (initial denaturation) |
35 cycles: | 10 sec | 98 (denaturation) |
30 sec | 60°C (annealing) | |
40 sec | 72°C (extension) | |
1 cycle: | 2 min | 72°C (final extension) |
Final step: | Cool down to | 4°C. |
9.Prepare a 1% agarose gel for the visualization of the PCR products as follows:
-
For 25 ml agarose gel, mix 25 ml TBE Buffer with 250 mg agarose in an Erlenmeyer flask.
-
Warm the mixture, e.g., in the microwave oven until all of the agarose is solved, avoid boil over.
-
Add a DNA visualizing reagent to the liquid gel (e.g., Midori Green) and pour it into a suitable gel chamber provided with a suitable gel comb.
-
Let the gel polymerize for about 20-30 min.
-
Transfer the gel into the electrophoresis chamber filled with TBE Buffer.
10.Load the PCR products with loading dye to the agarose gel and start the electrophoresis.
11.After electrophoresis, control if the size of the received DNA bands matches with the expected size of your desired PCR products.
COMMENTARY
Background Information
PSCs have versatile advantages; they permit in vitro studies, disease modeling, and therapeutic development. Differentiation of iPSCs into somatic cell types of interest is critical to the success of iPSC-based models. With the generation of PSC-derived reporter cell lines, developmental processes can be better understood and differentiation processes can be improved. For the derivation of those reporter PSCs, endogenous genomic loci have to be precisely edited with the help of programmable sequence-specific endonucleases such as zinc-finger nucleases (ZFNs) (Miller et al., 2007; Sander et al., 2011; Wood et al., 2011), transcription activator-like effector nucleases (TALENs) (Christian et al., 2010; Wood et al., 2011; Zhang et al., 2011), transposon-systems (Klompe, Vo, Halpin-Healy, & Sternberg, 2019; Querques et al., 2019; Voigt et al., 2016), or the RNA-guided CRISPR/Cas9 nuclease system (Cho, Kim, Kim, & Kim, 2013; Doudna & Charpentier, 2014). Cas9 is guided by small RNAs (gRNA) through Watson-Crick base pairing with target DNA to induce double-stranded breaks (DSBs). The Cas9 system is markedly easier to design than, e.g., TALENs or ZFNs, highly specific and efficient. The specificity of this system can even be improved by using mutated Cas9 nickases (Ran et al., 2013). With this mutated form of Cas9 only the teamwork of two Cas9n with different guide RNAs targeting opposite DNA-strands lead to staggered double-stranded breaks of target DNA and therefore off-target mutagenesis can be minimized. After Cas9 cleavage, two major cellular damage repair pathways get initiated: the error prone non-homologous end joining (NHEJ) or the homology-directed repair (HDR) pathway. Through the NHEJ process DSBs are re-ligated leaving insertion/deletion (indel) mutations. In the presence of an exogenous repair template, transgenes can be introduced by the cellular HDR repair pathway, which is generally active in dividing cells (Saleh-Gohari & Helleday, 2004)). In our protocol we describe the use of the CRISPR/Cas9n technology combined with the design and application of a polycistronic repair vector.
Critical Parameters and Troubleshooting
Quality of the PSCs
The health and quality of the PSCs are of critical importance for the process of cell clone derivation due to the stress coming with the transfection and selection of the cells. The number of passages has to be preferable low, as the genomic and phenotypic quality of PSCs deteriorates with the number of passages. The process of cell clone derivation will take a few passages and successfully edited cell clones should still have high quality for significant results. To maintain PSC viability, medium has to be replaced every day. When necessary, differentiated cells in the PSC culture must be removed manually.
Plasmid DNA quality for transfections
When a new PSC line is constructed, efficient transfection is crucial for successful gene editing. Higher rates of transfected cells will lead to higher possibilities of cell clones with the desired transgene insertion. The ideal concentration of plasmid DNA for transfection should be higher than 1 µg/µl. Midi- or Maxiprep DNA gives higher transfection rates than Miniprep DNA presumably due to lower bacterial endotoxin levels in bigger preparations. Also the quality of fresh plasmid DNA preparations tends to be better than of older preparations with several freeze-thaw cycles. Therefore, DNA for transfection should be relatively freshly prepared in Midi- or Maxipreparations.
Sterility and good cell culture practices
Cell culture of PSCs and the involved protocol steps should be performed under sterile conditions to avoid contaminations. Media and buffers need to be sterile and filtered through a 0.22-µm filter, if necessary. It is critical to avoid cross-contamination of the generated cell clones. Therefore, take care of each generated cell clone separately if possible.
Quality control of generated cell clones
It is critical for the functionality of the obtained cell clones to exclude mutations triggered by the editing process. Therefore, it is important to control the correct insertion of the transgenes via sequencing of the PCR-products (Basic Protocol 3). It is not less important to sequence also the wildtype-PCR product of heterozygous clones to avoid a knock-out of the target gene on the wildtype allele due to indel-mutations. If the sequencing results are alright and the visual observation of the cell clone in culture is within the expected range for pluripotent cells, functionality tests can be performed. In order to find the cell clone candidate with the best quality we recommend testing of several cell clones.
Understanding Results
The protocols provide detailed instructions for the derivation of pluripotent reporter stem cell lines. Basic Protocol 1 describes the design and cloning of the required CRISPR/Cas9n and the construction of polycistronic repair vectors for homology directed repair. With preparations of DNA of a CRISPR/Cas9n pair and a repair vector, pluripotent stem cells can be transfected. The transfection of pluripotent stem cells is described in Basic Protocol 2. It is important to develop a kill-curve with the required antibiotic for each cell line individually before starting with transfections. Also the proper magnitude and length of the electric pulse used for electroporation has to be determined for each cell line prior to transfection. If the transfection of the cells was successful, the obtained clonal cell colonies can be expanded (Basic Protocol 2) and screened for the correct transgene insertion. Depending on the cell line, gene locus and HDR template, we would expect editing efficiencies between 20% and 90%, so testing of 20-50 cell clones should give a sufficient number of positive clones. The design of primer pairs for wildtype test and confirmation of correct transgene insertion is provided in Basic Protocol 3. PCR products of wild-type PCR and transgene PCR should be sent for sequencing. After sequencing, functionality tests have to be done, including tests for transgene expression of reporter proteins, proper differentiation capacities compared to wild-type PSCs and more. Executing this protocol, there can still be variations of editing efficiencies. These variations can be traced back to several reasons. Some constructs work great in one gene locus, but in another one it can be difficult to obtain cell clones. Some stem cell lines are easier to transfect and edit than others, and also the length of the transgene construct plays a role, as smaller ones are often easier to introduce into a gene locus than bigger ones. It is difficult to give efficiency rates in general, because the success of editing PSCs depends on various factors.
Time Considerations
The repair vector and CRISPR/Cas9n design can be completed within a few days. When the required vectors and oligomers are ordered and delivered, the cloning process described in Basic Protocol 1 occupies 1-2 weeks. Transfection of PSCs described in Basic Protocol 2 can be done in approximately 1 hr. The following selection and expansion of cell clones takes 4-6 weeks depending on the growth rate of the cells. The genotyping of the generated cell clone described in Basic Protocol 3 can be completed within 1 week.
Acknowledgments
This work was funded by the German Research Foundation (NA-1285/2-1).
Literature Cited
- Bothmer, A., Phadke, T., Barrera, L. A., Margulies, C. M., Lee, C. S., Buquicchio, F., … Cotta-Ramusino, C. (2017). Characterization of the interplay between DNA repair and CRISPR/Cas9-induced DNA lesions at an endogenous locus. Nature Communications , 8, 13905. doi: 10.1038/ncomms13905.
- Brees, C., & Fransen, M. (2014). A cost-effective approach to microporate mammalian cells with the Neon Transfection System. Analytical Biochemistry , 466, 49–50. doi: 10.1016/j.ab.2014.08.017.
- Cho, S. W., Kim, S., Kim, J. M., & Kim, J. S. (2013). Targeted genome engineering in human cells with the Cas9 RNA-guided endonuclease. Nature Biotechnology , 31(3), 230–232. doi: 10.1038/nbt.2507.
- Christian, M., Cermak, T., Doyle, E. L., Schmidt, C., Zhang, F., Hummel, A., … Voytas, D. F. (2010). Targeting DNA double-strand breaks with TAL effector nucleases. Genetics , 186(2), 757–761. doi: 10.1534/genetics.110.120717.
- Cong, L., Ran, F. A., Cox, D., Lin, S., Barretto, R., Habib, N., …Zhang, F. (2013). Multiplex genome engineering using CRISPR/Cas systems. Science , 339(6121), 819–823. doi: 10.1126/science.1231143.
- Doudna, J. A., & Charpentier, E. (2014). Genome editing. The new frontier of genome engineering with CRISPR-Cas9. Science , 346(6213), 1258096. doi: 10.1126/science.1258096.
- Hartman, M. E., Librande, J. R., Medvedev, I. O., Ahmad, R. N., Moussavi-Harami, F., Gupta, P. P., … Chin, M. T. (2014). An optimized and simplified system of mouse embryonic stem cell cardiac differentiation for the assessment of differentiation modifiers. Plos One , 9(3), e93033. doi: 10.1371/journal.pone.0093033.
- Klompe, S. E., Vo, P. L. H., Halpin-Healy, T. S., & Sternberg, S. H. (2019). Transposon-encoded CRISPR-Cas systems direct RNA-guided DNA integration. Nature , 571(7764), 219–225. doi: 10.1038/s41586-019-1323-z.
- Liu, Z., Chen, O., Wall, J. B. J., Zheng, M., Zhou, Y., Wang, L., … Liu, J. (2017). Systematic comparison of 2A peptides for cloning multi-genes in a polycistronic vector. Scientific Reports , 7(1), 2193. doi: 10.1038/s41598-017-02460-2.
- Mali, P., Aach, J., Stranges, P. B., Esvelt, K. M., Moosburner, M., Kosuri, S., … Church, G. M. (2013). CAS9 transcriptional activators for target specificity screening and paired nickases for cooperative genome engineering. Nature Biotechnology , 31(9), 833–838. doi: 10.1038/nbt.2675.
- Miller, J. C., Holmes, M. C., Wang, J., Guschin, D. Y., Lee, Y. L., Rupniewski, I., … Rebar, E. J. (2007). An improved zinc-finger nuclease architecture for highly specific genome editing. Nature Biotechnology , 25(7), 778–785. doi: 10.1038/nbt1319.
- Missirlis, P. I., Smailus, D. E., & Holt, R. A. (2006). A high-throughput screen identifying sequence and promiscuity characteristics of the loxP spacer region in Cre-mediated recombination. BMC Genomics , 7, 73. doi: 10.1186/1471-2164-7-73.
- Querques, I., Mades, A., Zuliani, C., Miskey, C., Alb, M., Grueso, E., … Barabas, O. (2019). A highly soluble Sleeping Beauty transposase improves control of gene insertion. Nature Biotechnology , 37(12), 1502–1512. doi: 10.1038/s41587-019-0291-z.
- Ran, F. A., Hsu, P. D., Lin, C. Y., Gootenberg, J. S., Konermann, S., Trevino, A. E., … Zhang, F. (2013). Double nicking by RNA-guided CRISPR Cas9 for enhanced genome editing specificity. Cell , 154(6), 1380–1389. doi: 10.1016/j.cell.2013.08.021.
- Saleh-Gohari, N., & Helleday, T. (2004). Conservative homologous recombination preferentially repairs DNA double-strand breaks in the S phase of the cell cycle in human cells. Nucleic Acids Research , 32(12), 3683–3688. doi: 10.1093/nar/gkh703.
- Sander, J. D., Dahlborg, E. J., Goodwin, M. J., Cade, L., Zhang, F., Cifuentes, D., … Joung, J. K. (2011). Selection-free zinc-finger-nuclease engineering by context-dependent assembly (CoDA). Nature Methods , 8(1), 67–69. doi: 10.1038/nmeth.1542.
- Sternberg, N., Hamilton, D., & Hoess, R. (1981). Bacteriophage P1 site-specific recombination. II. Recombination between loxP and the bacterial chromosome. Journal of Molecular Biology , 150(4), 487–507. doi: 10.1016/0022-2836(81)90376-4.
- Szymczak, A. L., Workman, C. J., Wang, Y., Vignali, K. M., Dilioglou, S., Vanin, E. F., & Vignali, D. A. (2004). Correction of multi-gene deficiency in vivo using a single ‘self-cleaving’ 2A peptide-based retroviral vector. Nature Biotechnology , 22(5), 589–594. doi: 10.1038/nbt957.
- Torres, V., Barra, L., Garces, F., Ordenes, K., Leal-Ortiz, S., Garner, C. C., … Zamorano, P. (2010). A bicistronic lentiviral vector based on the 1D/2A sequence of foot-and-mouth disease virus expresses proteins stoichiometrically. Journal of Biotechnology , 146(3), 138–142. doi: 10.1016/j.jbiotec.2010.01.017.
- Veres, A., Faust, A. L., Bushnell, H. L., Engquist, E. N., Kenty, J. H., Harb, G., … Melton, D. A. (2019). Charting cellular identity during human in vitro beta-cell differentiation. Nature , 569(7756), 368–373. doi: 10.1038/s41586-019-1168-5.
- Vieyra, D. S., & Goodell, M. A. (2007). Pluripotentiality and conditional transgene regulation in human embryonic stem cells expressing insulated tetracycline-ON transactivator. Stem Cells , 25(10), 2559–2566. doi: 10.1634/stemcells.2007-0248.
- Voigt, F., Wiedemann, L., Zuliani, C., Querques, I., Sebe, A., Mates, L., … Barabas, O. (2016). Sleeping Beauty transposase structure allows rational design of hyperactive variants for genetic engineering. Nature Communications , 7, 11126. doi: 10.1038/ncomms11126.
- Wolf, D., & Goff, S. P. (2007). TRIM28 mediates primer binding site-targeted silencing of murine leukemia virus in embryonic cells. Cell , 131(1), 46–57. doi: 10.1016/j.cell.2007.07.026.
- Wood, A. J., Lo, T. W., Zeitler, B., Pickle, C. S., Ralston, E. J., Lee, A. H., … Meyer, B. J. (2011). Targeted genome editing across species using ZFNs and TALENs. Science , 333(6040), 307. doi: 10.1126/science.1207773.
- Zhang, F., Cong, L., Lodato, S., Kosuri, S., Church, G. M., & Arlotta, P. (2011). Efficient construction of sequence-specific TAL effectors for modulating mammalian transcription. Nature Biotechnology , 29(2), 149–153. doi: 10.1038/nbt.1775.
Internet Resource
Online tool CCTOP for the determination of suitable target sequences for CRISPR/Cas9n.
Citing Literature
Number of times cited according to CrossRef: 2
- Javier Fierro, Jake DiPasquale, Joshua Perez, Brandon Chin, Yathip Chokpapone, An M. Tran, Arabella Holden, Chris Factoriza, Nikhi Sivagnanakumar, Rocio Aguilar, Sarah Mazal, Melissa Lopez, Huanyu Dou, Dual-sgRNA CRISPR/Cas9 knockout of PD-L1 in human U87 glioblastoma tumor cells inhibits proliferation, invasion, and tumor-associated macrophage polarization, Scientific Reports, 10.1038/s41598-022-06430-1, 12 , 1, (2022).
- Rabea Dettmer, Isabell Niwolik, Ilir Mehmeti, Anne Jörns, Ortwin Naujok, New hPSC SOX9 and INS Reporter Cell Lines Facilitate the Observation and Optimization of Differentiation into Insulin-Producing Cells, Stem Cell Reviews and Reports, 10.1007/s12015-021-10232-9, 17 , 6, (2193-2209), (2021).