Validating TBMs by Fluorescence Polarization (FP)
Katie Pollock, Michael Ranes, Ian Collins, Sebastian Guettler
Tankyrase
PARP
Poly(ADP-ribosyl)ation
Tankyrase-binding peptide motif
Enzyme–substrate relationships
Protein-protein interactions
Protein expression
Protein purification
Fluorescence polarization
FP
Structural biology
Abstract
This protocol is part of a collection: Identifying and Validating Tankyrase Binders and Substrates: A Candidate Approach
The poly(ADP-ribose)polymerase (PARP) enzyme tankyrase (TNKS/ARTD5, TNKS2/ARTD6) uses its ankyrin repeat clusters (ARCs) to recognize degenerate peptide motifs in a wide range of proteins, thereby recruiting such proteins and their complexes for scaffolding and/or poly(ADP-ribosyl)ation. Here, we provide guidance for predicting putative tankyrase-binding motifs, based on the previously delineated peptide sequence rules and existing structural information. We present a general method for the expression and purification of tankyrase ARCs from Escherichia coli and outline a fluorescence polarization assay to quantitatively assess direct ARC–TBM peptide interactions. We provide a basic protocol for evaluating binding and poly(ADP-ribosyl)ation of full-length candidate interacting proteins by full-length tankyrase in mammalian cells.
Before start
Steps
Expression of Tankyrase ARCs
Transform chemically competent BL21-CodonPlus (DE3)-RIL E. coli cells with tankyrase ARC expression constructs by heat shock. Plate cells on LB agar plates containing suitable antibiotics, here 50microgram per milliliter (μg/mL)
and 34microgram per milliliter (μg/mL)
. Incubate at 37°C
.
Pick a single colony from the plate and inoculate an overnight pre-culture of 100mL
supplemented with 50microgram per milliliter (μg/mL)
and 34microgram per milliliter (μg/mL)
. Incubate in a shaking incubator at 37°C
.
Add 5mL
to each liter of TB medium supplemented with 50microgram per milliliter (μg/mL)
and 34microgram per milliliter (μg/mL)
. Generally, the yield for individual TNKS and TNKS2 ARC constructs is 6–10 mg/L of culture.
Incubate cultures at 37°C
with shaking at 180rpm
until an optical density at 600 nm (OD600) of approximately 2.0 is reached. Remove cultures from incubator and cool them at 4°C
for 0h 30m 0s
.
Add IPTG to a final concentration of 0.5millimolar (mM)
(0.5 mL of 1 M stock per liter of culture) to induce protein production. Incubate cultures at 18°C
with shaking 0h 30m 0s
(≈15 h).
Collect cells by centrifugation in a precooled centrifuge at 4000x g,4°C
.
Discard supernatant. The cells can be lysed for immediate purification. Alternatively, pellets can be flash-frozen in a liquid nitrogen bath for storage at -80°C
until required .
3.2.2 Purification of Tankyrase ARCs
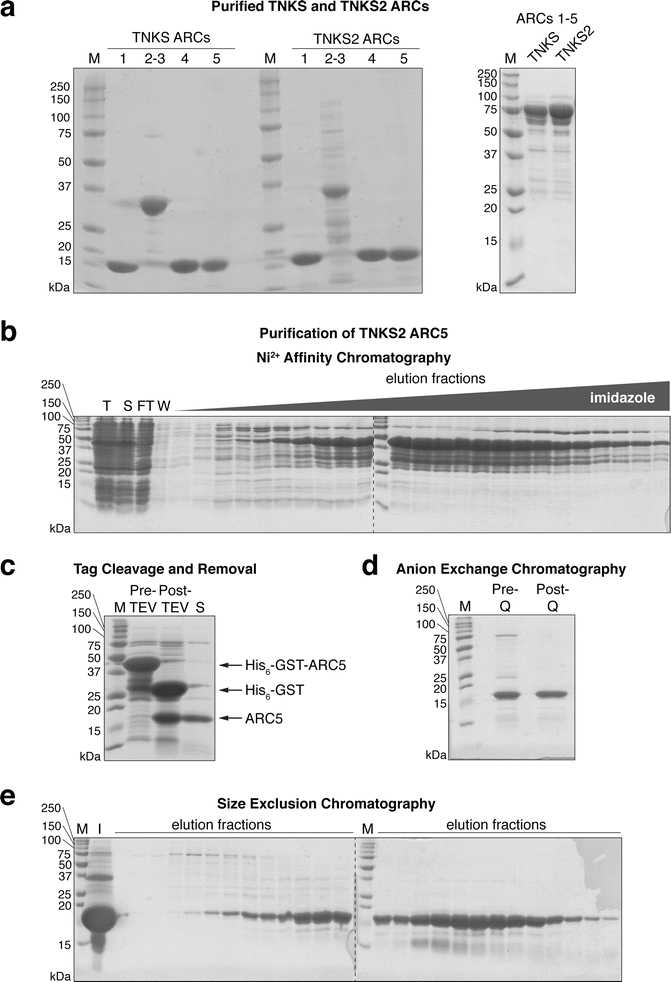
Dissolve protease inhibitor tablets in the required volume of lysis buffer (approximately 20mL
for every 10g
) and add lysozyme (100microgram per milliliter (μg/mL)
final) in a large glass beaker containing a magnetic stirrer bar. Add frozen cell pellet and stir gently until cells are completely thawed and a homogeneous suspension is obtained.
Break cells by using a large-tip sonicator at 40% amplitude output, using a 0h 0m 5s
on, 0h 0m 5s
off pulse, for a total of 0h 5m 0s
. Keep cells On ice
continuously to avoid overheating. Take a sample of the total lysate for subsequent analysis by SDS-PAGE (9µL
+ 3µL
).
Remove cell debris and insoluble material by centrifugation at 30000x g
. If lysate is still cloudy, centrifuge for a further 0h 30m 0s
until clear.
While the lysate is being cleared, prepare a 5mL
by washing with 10column volumes (CV)
and then equilibrating with 10column volumes (CV)
for Ni2+ affinity column, using a low-pressure peristaltic pump, set to a flow rate of 2 mL/min.
Decant the supernatant into a beaker, and sonicate On ice
, 0h 0m 5s
on, 0h 0m 5s
off for a total of 0h 0m 20s
to further break up genomic DNA and avoid clogging the filter in the next step.
Filter the supernatant through 5.0 μm syringe filter units. Take a sample of the soluble lysate for subsequent analysis by SDS-PAGE (9µL
+ 3µL
). Load the cleared lysate on the equilibrated HisTrap column using the peristaltic pump as before.
The His6-GST-tagged protein will bind to the immobilized Ni2+ ions. Wash the column with 20column volumes (CV)
to remove unbound contaminants. Collect lysate flow-through and the wash buffer in case the protein was not bound or was released during the wash, and take samples for analysis by SDS-PAGE (9µL
+ 3µL
).
Attach the HisTrap column to an FPLC system, and elute the His6-GST-ARC protein with the following three-step protocol, at a flow rate of 2 mL/min, collecting 1.5 mL fractions in a 96-deep-well block:
step 1: 2column volumes (CV)
.
step 2: over 20column volumes (CV)
.
step 3: 4column volumes (CV)
.
Take samples of each peak elution fraction (9µL
+ 3µL
). Analyze the samples taken in steps 11 and 15 (2 μL of sample for lysates and flow-through, 5µL
for wash) and the peak elution fractions (5µL
) by SDS-PAGE (Fig. 2b).
Pool fractions containing ARC fusion protein. Take a sample of the pooled fractions (9µL
+ 3µL
). Add TEV protease (approximately 100µL
for every 25mL
) to cleave the tag.
Soak dialysis tubing (3500 Da MWCO) in dialysis buffer, and seal one end with a dialysis membrane clip. Transfer the protein into the dialysis tubing and seal the other end. More than one dialysis tube may be required. Dialyze 0h 0m 20s
in a 2 L glass beaker containing dialysis buffer, with gentle stirring at 4°C
. Avoid contact between the stirrer bar and the dialysis tubing to prevent rupture.
Pour content of dialysis tubing into 50 mL Falcon tubes. Centrifuge at 3200x g
to remove any precipitate that may have formed. Take a sample of the protein post-TEV cleavage (9µL
+ 3µL
). White precipitate often forms overnight with the change to low-salt buffer. SDS-PAGE analysis of the precipitate reveals it to be enriched in a contaminant (not shown).
Meanwhile, wash a 5mL
with 10column volumes (CV)
. Next, equilibrate the column in 10column volumes (CV)
. Filter the supernatant through a 0.22 μm syringe filter and load it onto the equilibrated HisTrap column using the low-pressure peristaltic pump. Collect the flow-through. The untagged ARC protein will pass through, while the cleaved tag (or residual His6-GST-ARC fusion protein) will be retained on the column.
9µL
+ 3µL
). Analyze 5µL
, post-TEV sample and flow-through/wash samples from the second Ni2+ column by SDS-PAGE to assess successful tag removal and separation (Fig. 2c).
Take the protein-containing samples (flow-through/wash) and concentrate the protein to about 20mL
by centrifuging in a 3000 Da MWCO spin concentrator at 3200x g
at a time in a swinging bucket rotor. Mix by gently pipetting up and down to prevent a steep concentration gradient from forming in the concentrator. Take a sample of the concentrated protein (9µL
+ 3µL
).
The following anion exchange chromatography step is optional, but was found to increase the purity of ARC proteins, especially TNKS2 ARC5, by removing a higher-molecular-weight impurity:
Using the peristaltic pump at 2 mL/min , wash a 5 mL HiTrap Q HP column with 5column volumes (CV)
, followed by 5column volumes (CV)
for Q column, and then equilibrate with 10column volumes (CV)
for Q column. Pass the concentrated protein over the column, and collect the flow-through. Wash the column with 2column volumes (CV)
for Q column, also collecting the flow-through. ARCs do not bind to the Q column; however, the impurity does and will be retained on the column.
Take a sample of the combined flow-through and wash (9µL
+ 3µL
). Analyze 5µL
from step 23 and this step by SDS-PAGE as above (Fig. 2d).
Concentrate the protein as in step 23, to ≤2mL
: Take the protein-containing samples (flow-through/wash) and concentrate the protein by centrifuging in a 3000 Da MWCO spin concentrator at 3200x g
at a time in a swinging bucket rotor. Mix by gently pipetting up and down to prevent a steep concentration gradient from forming in the concentrator. Take a sample of the concentrated protein (9µL
+ 3µL
).
Centrifuge protein in a microcentrifuge tube at 18000x g
to remove any particles before size exclusion chromatography.
Load the concentrated protein onto an equilibrated size exclusion column connected to an FPLC system , using a 2 mL sample loop.
1.2column volumes (CV)
. Collect 1 mL fractions in a 96-deep-well block. Take samples of the input material and peak fractions (9µL
or 9µL
+ 3µL
). Analyze 5µL
by SDS-PAGE (Fig. 2e).
Combine fractions containing pure protein. Wash the membrane of a spin concentrator by spinning through one concentrator volume of H2O to remove any stabilizing agents such as glycerol and azide. Now concentrate the protein using the pre-washed spin concentrator as described above (step 24) until a concentration of >0.5millimolar (mM)
is achieved (solubility of multi- ARC constructs can be lower). The concentration can be determined by measuring the UV absorbance at 280 nm and using the molar extinction coefficient calculated by ProtParam (http://web.expasy.org/protparam/) [29].
Aliquot the protein into small volumes in thin-walled PCR tubes and flash-freeze them in a liquid nitrogen bath. Frozen protein aliquots can be stored at -80°C
until future use.
3.2.3 Fluorescence Polarization Assay for TBM Validation
Ensure the plate reader is set up for fluorescence polarization, with the correct filters for the fluorophore . The fluorescein example uses a 485 nm excitation filter and two 520 nm emission filters, one parallel and one perpendicular to the plane of the linearly polarized light used for excitation.
Dissolve the lyophilized peptide probe in FP assay buffer, to approximately 100micromolar (µM)
. The concentration can be measured spectrophotometrically.
Here, we measure the absorbance of the peptide stock at 492 nm and use a molar extinction coefficient for fluorescein of ε492 = 83,000/M/cm [30]. The concentrated probe can then be aliquoted into small volumes (5–10 μL) and flash-frozen in liquid nitrogen for long-term storage at -80°C
, to be thawed when required.
Determine the optimal probe concentration to use. This will depend on the sensitivity of the plate reader. FP values should be independent of the probe concentration used, and give a robust signal window for an unbound vs. protein-bound peptide. Make 2× stocks of a twofold dilution series of probe in FP buffer, from 0–800 nM (10 concentrations + no peptide). It is often easier to make a 1/100 dilution of the concentrated peptide stock to avoid pipetting small volumes. Total sample volumes per well are either 15 or 50 μL (see step 36 below).
Prepare a 2× protein stock solution, by diluting tankyrase ARC protein in FP buffer to 20micromolar (µM)
(10 μM final protein concentration). The protein concentration should be in vast excess over the peptide probe to obtain a first impression of the anticipated signal window between free and protein-bound peptide probe .
Plate out the assay samples in technical duplicate (see Table 2 and note below). Small-volume plates will require 15 μL per well (7.5µL
of each stock), standard-volume plates 50µL
per well (25µL
of each stock). Mix the probe stock solution 1:1 with either FP buffer for the peptide probe-only titration or protein stock for the titration in the presence of protein. Add a “blank” well with FP buffer only. A well with 5nanomolar (nM)
(literature FP of 35 millipolarization units, mP) can be used as a standard to automatically set the gain adjustment of the plate reader. Temporarily seal the plate with adhesive film or a lid and spin at 1000x g
in a swinging bucket rotor with plate inserts. Incubate in the dark at 80Room temperature
for 0h 30m 0s
to ensure binding equilibrium is reached.
A | B | C | D | E | F | G | H | I | J | K | L | M | N | O |
---|---|---|---|---|---|---|---|---|---|---|---|---|---|---|
1 | 2 | 3 | 4 | 5 | 6 | 7 | 8 | 9 | 10 | 11 | 12 | 13 | 14 | |
A | ||||||||||||||
B | 0 | 0.78 | 1.56 | 3.13 | 6.25 | 12.5 | 25 | 50 | 100 | 200 | 400 | Blank | Standard | |
C | 0 | 0.78 | 1.56 | 3.13 | 6.25 | 12.5 | 25 | 50 | 100 | 200 | 400 | Blank | Standard | |
D | 0 | 0.78 | 1.56 | 3.13 | 6.25 | 12.5 | 25 | 50 | 100 | 200 | 400 | Blank | Standard | |
E | 0 | 0.78 | 1.56 | 3.13 | 6.25 | 12.5 | 25 | 50 | 100 | 200 | 400 | Blank | Standard |
Table 2Example plate layout for establishing the probe concentration in a fluorescence polarization assay. Rows B and C correspond to 3BP2 peptide titrations (here up to 400 nM) in the absence of protein, while rows D and E correspond to 3BP2 peptide titrations in the presence of protein. Numbers correspond to final fluorophore concentration (nM). Blank wells contain buffer alone. Standard wells contain 5 nM of free fluorescein. Wells along the edge of the microplate (row A and column 1) are left empty to minimize the “edge effect”
Set the gain adjustment of the instrument. The POLARstar Omega automatically calculates the gain by scaling the FP value to 35 mP for the wells containing 5nanomolar (nM)
. 50 flashes per well is a good starting point to minimize fluorophore bleaching, and means each data point will be the average of 50 measurements.
Read the plate and calculate FP in mP. Some plate readers and software packages will automatically blank-correct and calculate the FP values. If not, subtract the averaged F_parallel and F_perpendicular readings obtained for the blank wells from the corresponding individual values of the other wells. FP values are calculated using the following equation:
F_parallel and F_perpendicular denote the fluorescence intensities parallel and perpendicular to the plane of the linearly polarized lightused for excitation. mP, millipolarization units.
Export the FP data and raw fluorescence intensity values. Plot the data (average FP vs. [probe]) using GraphPad Prism or your data analysis program of choice (Fig.3a).
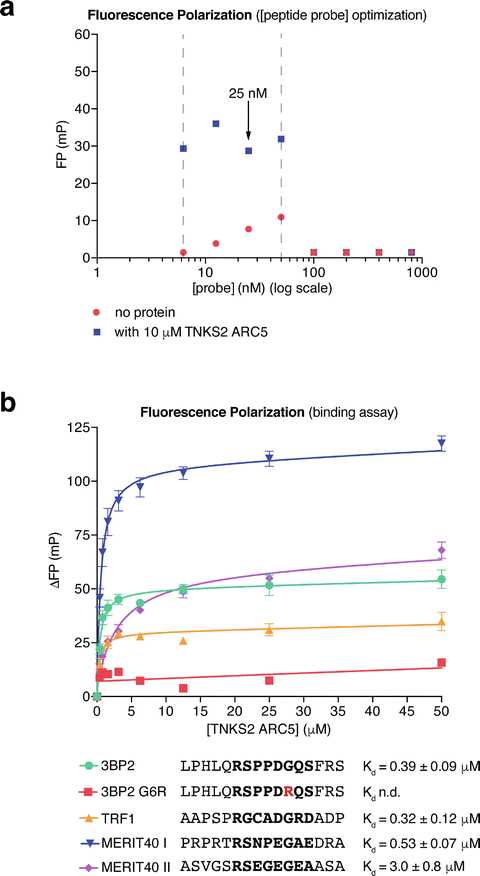
Determine the minimum probe concentration required. Raw fluorescence intensity values of >10× the blank reading are necessary to calculate accurate FP values. In the example shown in Fig. 3a, fluorescence intensity values measured with probe concentrations below 6.25nanomolar (nM)
were too low and therefore excluded.
Determine the maximum probe concentration. The FP values will drop sharply when the signal overloads the detectors. In this case, both detectors reach saturation, so the fluorescence intensities registered in the parallel and perpendicular channels will be identical, and hence the FP calculated will be zero. In the example shown in Fig. 3a, 50nanomolar (nM)
is the maximum usable peptide probe concentration. FP readings between 6.25 and 50 nM probe are approximately constant.
Peptide probe concentrations should be kept as low as reasonably possible. Thus, the peptide concentration can be ignored in Kdcalculations. 25 nM probe was chosen for the protein titration experiments shown below. Prepare a 2× probe stock solution (50nanomolar (nM)
in FP buffer). Prepare a twofold dilution series of tankyrase ARC protein from 0–400 μM (0–200 μM final concentration) in FP buffer. The maximum protein concentration may be lowered if B max is reached at low protein concentrations, i.e., in the case of high affinity. An initial maximum [ARC] of 200micromolar (µM)
ensures that binding can be detected for weak binders as well.
Set up a binding experiment for Kddetermination. Mix the probe and protein stock solution 1:1 to give 15µL
(7.5 μL of each stock solution, small-volume plates). Centrifuge the plate as in step 39 (spin at 1000x g
in a swinging bucket rotor with plate inserts) above. Incubate the plate in the dark at 80Room temperature
for 0h 30m 0s
to achieve equilibrium before measurement.
Calculate FP as in step 38 above and export the raw fluorescence and calculated FP values.
Enter the individual FP values into GraphPad Prism or your data analysis program of choice.
Baseline-correct by subtracting the FP value for the zero-protein well from all other values in that row, to obtain ΔFP values. Calculate the mean of the technical duplicates. Plot ΔFP against [protein]. Perform a nonlinear regression analysis, using a one-site total binding model:
Bmax denotes the maximum specific binding in mP, where the binding curve saturates; K d is the dissociation constant ; NS denotes the slope of nonspecific binding in ΔFP/[ARC] units; Background denotes the baseline ΔFP value, which should be zero. Although it is possible to constrain the background to zero, it is advisable to retain the background term since the baseline is defined by more values than that from the zero-protein sample. maxdenotes the maximum specific binding in mP, where the binding curve saturates; K d is the dissociation constant ; NS denotes the slope of nonspecific binding in ΔFP/[ARC] units; Background denotes the baseline ΔFP value, which should be zero. Although it is possible to constrain the background to zero, it is advisable to retain the background term since the baseline is defined by more values than that from the zero-protein sample.
Non-binding peptides will give a straight line of nonspecific binding. Binding peptides will give a logarithmic curve (Fig. 3b). This analysis will yield a Kd value.
Repeat the experiment to obtain pooled data from (typically three) separate experiments . In each separate experiment, technical duplicates are combined into a single data point. Error bars (SEM) are calculated for the mean data points from the separate experiments (Fig. 3b). The peptides from 3BP2 , TRF1 , and MERIT40 (two peptides) bind TNKS2 ARC5 with the indicated affinities, while no binding is detectable for the G6R negative control peptide from 3BP2 (Fig. 3b).