Orsay Virus Infection in Caenorhabditis elegans
Lakshmi E. Batachari, Lakshmi E. Batachari, Mario Bardan Sarmiento, Mario Bardan Sarmiento, Nicole Wernet, Nicole Wernet, Emily R. Troemel, Emily R. Troemel
Abstract
Orsay virus infection in the nematode Caenorhabditis elegans presents an opportunity to study host-virus interactions in an easily culturable, whole-animal host. Previously, a major limitation of C. elegans as a model for studying antiviral immunity was the lack of viruses known to naturally infect the worm. With the 2011 discovery of the Orsay virus, a naturally occurring viral pathogen, C. elegans has emerged as a compelling model for research on antiviral defense. From the perspective of the host, the genetic tractability of C. elegans enables mechanistic studies of antiviral immunity while the transparency of this animal allows for the observation of subcellular processes in vivo. Preparing infective virus filtrate and performing infections can be achieved with relative ease in a laboratory setting. Moreover, several tools are available to measure the outcome of infection. Here, we describe workflows for generating infective virus filtrate, achieving reproducible infection of C. elegans , and assessing the outcome of viral infection using molecular biology approaches and immunofluorescence. © 2024 The Authors. Current Protocols published by Wiley Periodicals LLC.
Basic Protocol 1 : Preparation of Orsay virus filtrate
Support Protocol : Synchronize C. elegans development by bleaching
Basic Protocol 2 : Orsay virus infection
Basic Protocol 3 : Quantification of Orsay virus RNA1/RNA2 transcript levels by qRT-PCR
Basic Protocol 4 : Quantification of infection rate and fluorescence in situ hybridization (FISH) fluorescence intensity
Basic Protocol 5 : Immunofluorescent labeling of dsRNA in virus-infected intestinal tissue
INTRODUCTION
Isolated from wild strains of Caenorhabditis elegans , the Orsay virus is the only natural virus known to infect this nematode (Félix & Wang, 2019; Félix et al., 2011). Belonging to the Nodaviridae family, the Orsay virus is a positive-sense, single-stranded RNA virus with a genome that consists of two segments, RNA1 and RNA2. At ∼3.4 kb, the RNA1 segment contains a gene that encodes the RNA-dependent RNA polymerase (RdRP), which controls viral replication. The 2.6 kb RNA2 segment encodes the viral capsid protein as well as a capsid-delta fusion protein (Jiang et al., 2014). Viral replication occurs within intestinal cells, and horizontal transmission from animal-to-animal enables viral spread; no vertical transmission has yet been reported for Orsay virus. As many canonical defense factors are absent in C. elegans , the study of immune responses in this nematode host has the potential to uncover novel antiviral defense pathways.
Several factors make C. elegans a powerful model for investigating cell-intrinsic as well as systemic (tissue-to-tissue) immune responses to viral infection in an intact animal. With a body plan of ∼1000 cells, C. elegans has a fully described invariant cell (Corsi et al., 2018). Facilitated by the small size of the organism, a fast generation time, and selfing hermaphrodite reproduction, C. elegans genetics enables high-throughput screening and mechanistic studies. The field also has an international, collaborative community of researchers with well-developed protocols, genetic tools, and the Caenorhabditis Genetics Center that catalogues and distributes wild-type and mutant strains to investigators. The C. elegans model also provides specific strengths to the study of intestinal immunity. For example, C. elegans and mammals share similarities in intestinal cell structure and function, increasing the potential for translational studies (Dimov & Maduro, 2019; Pukkila-Worley & Ausubel, 2012). The intestinal tissue tropism of the Orsay virus enables investigations of the mechanisms that shape intestinal immunity against viral pathogens. Moreover, the absence of known professional immune cells (e.g., macrophages, dendritic cells, T cells, B cells) presents an opportunity to explore how non-professional immune cells, including epithelial cells, coordinate organismal defense against a natural viral pathogen.
Fluorescent labeling of viruses offers a method to assess viral infection levels with spatial and temporal resolution. Unfortunately, it has not yet been possible to genetically encode a fluorescent tag within the Orsay virus genome, which would enable live labeling of the virus. Therefore, current methods for measuring the Orsay virus rely on fixation and fluorescence in situ hybridization (FISH) (Rivera et al., 2022). Although not possible in vivo, FISH labeling provides robust spatial and temporal resolution to assess viral infection. In addition to FISH, qRT-PCR has been used across several laboratories to measure viral load and/or infection rate (Jiang et al., 2014, 2017, 2020; Le Pen et al., 2018; Sandoval et al., 2019; Sowa et al., 2020). Immunofluorescence has also enabled the detection of viral proteins (Franz et al., 2014). However, standardized approaches for performing and measuring infection have not been described in detail. Here, we provide a centralized resource that outlines methods for infecting C. elegans with the Orsay virus (Basic Protocols 1 and 2) and quantifying the outcome of viral infection in a reproducible manner (Basic Protocols 3 and 4).
A distinctive feature of single-stranded RNA virus replication is the generation of double-stranded RNA (dsRNA) intermediates. Importantly, dsRNA is considered a ‘non-self’ molecule by the immune system and thus can serve as a key trigger of innate immune responses in mammalian cells (Chen & Hur, 2022), where immunofluorescence can be used to detect dsRNA (Son et al., 2015). A key advantage of C. elegans is that immunofluorescence can be used to assess subcellular components in intact animals. Here, we provide a detailed approach that combines FISH with immunofluorescence to simultaneously visualize viral RNA and dsRNA within adult intestinal cells (Basic Protocol 5).
With a biomass equivalent to 82% of total human biomass, nematodes are among the most abundant organisms on this planet (van den Hoogen et al., 2019), yet we have a relatively limited understanding of their antiviral defenses. Findings from multiple laboratories have demonstrated a role for RNA interference (RNAi) in antiviral defense in C. elegans (Ashe et al., 2013; Félix et al., 2011; Gammon, 2017; Guo et al., 2013; Lu et al., 2009). For example, loss-of-function mutations in the RNAi component RDE-1 results in increased susceptibility to virus. Independent of RNAi-mediated defense, a C. elegans terminal uridylyltransferase, CDE-1, promotes antiviral activity through uridylation and subsequent degradation of the Orsay virus genome (Le Pen et al., 2018). Orsay virus infection also induces an antiviral transcriptional immune response, termed the Intracellular Pathogen Response (IPR), that is distinct from RNAi (Bakowski et al., 2014; Reddy et al., 2017; Sowa et al., 2020). However, the relative contributions of these different pathways to antiviral defense remain unclear. We also have a limited understanding of the spatial relationships between host and viral proteins. To address these outstanding questions, the application of the protocols described below will enable further investigation of the C. elegans response to viral infection.
In this article, we describe five main protocols to facilitate studies of Orsay virus infection in C. elegans (Fig. 1). Basic Protocol 1 provides detailed steps to generate a large batch of Orsay virus filtrate for use in infection assays. Basic Protocol 2 describes a method for performing viral infections in synchronized first and fourth larval stage (L1 and L4) animals, although all post-embryonic stages of C. elegans are susceptible to infection. Basic Protocols 3 and 4 provide standardized approaches to assess the outcome of infection using qRT-PCR and FISH. Finally, Basic Protocol 5 describes steps to combine FISH and immunofluorescence to simultaneously visualize viral RNA and dsRNA in intestinal tissue.
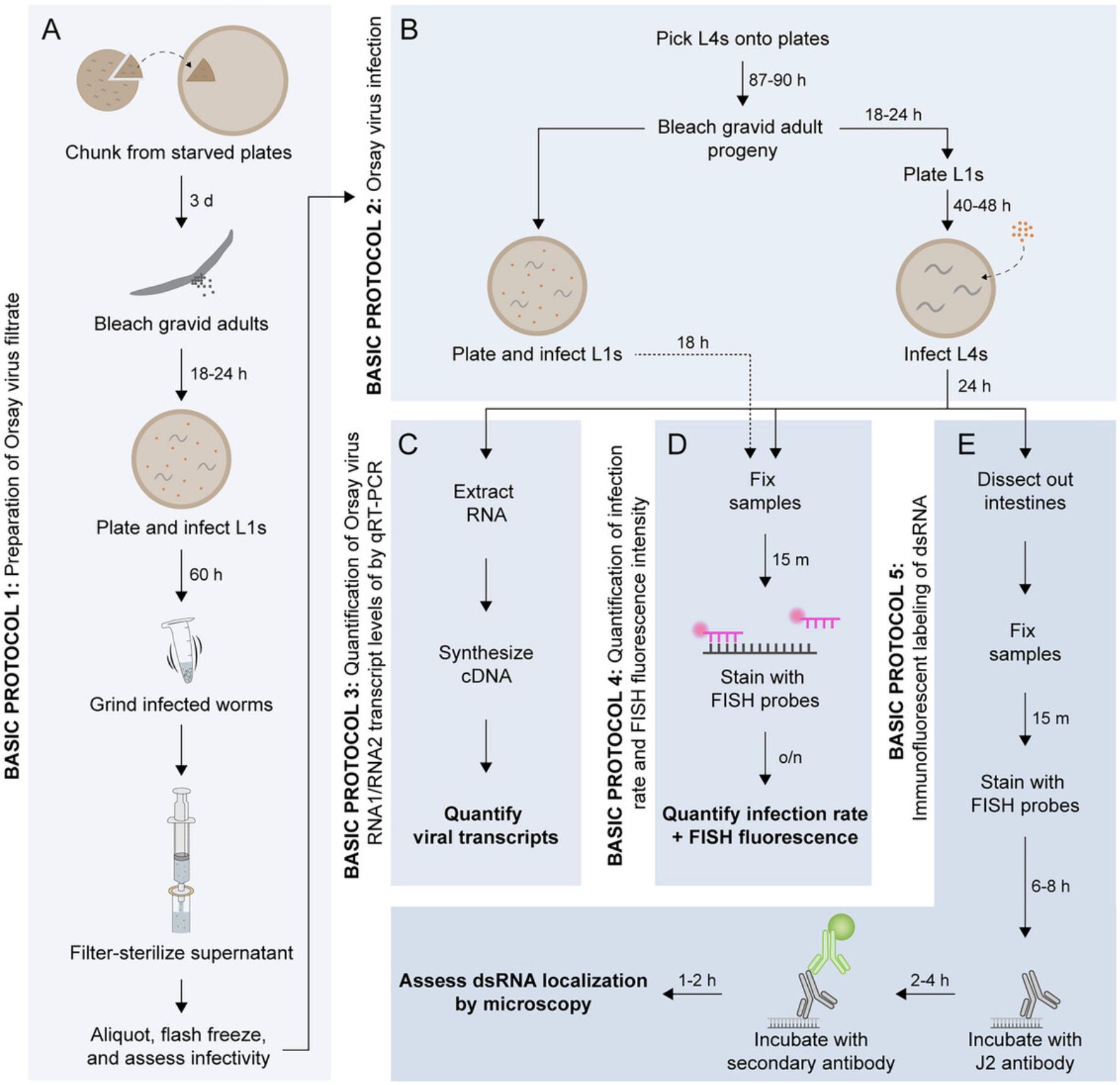
Basic Protocol 1: PREPARATION OF ORSAY VIRUS FILTRATE
Because C. elegans is the only known host of the Orsay virus, and no tissue culture systems exist for this host, the virus must be fed to and amplified inside of C. elegans animals that are grown on agar plates seeded with an Escherichia coli food source. As an alternate to agar plates, infected worms can be grown in liquid culture to amplify virus (Jiang et al., 2014). After amplification within C. elegans , virus filtrate can be extracted, aliquoted, and flash frozen in large batches to ensure reproducible infection across experiments (Fig. 1A). To increase the total number of viral particles generated, filtrate preparations are best performed in animals defective in RNAi and thus exhibiting higher viral load compared to N2 animals. For example, our lab uses RNAi-defective rde-1 mutant animals. Virus filtrate preparations can also be performed in animals that express the pals-5p ::GFP reporter (as part of the integrated transgene jyIs8). A member of the IPR gene family, pals-5 is highly upregulated during viral infection. Therefore, the pals-5p ::GFP reporter provides a visual indicator of infection that can be useful when preparing virus filtrate and determining an optimal viral titer for downstream assays.
Materials
-
C. elegans strains jyIs8 and/or rde-1; jyIs8 (Caenorhabditis Genetics Center)
-
Standard 6-well, 6- and 10-cm Nematode Growth Medium (NGM) plates seeded with E. coli OP50-1 (Stiernagle, 2006)
-
Bleaching solution (see Support Protocol)
-
M9 buffer (see recipe)
-
Orsay virus filtrate (OVF)
-
To obtain virus, contact: Emily Troemel, etroemel@ucsd.edu; Marie-Anne Felix, felix@bio.ens.psl.eu; or Dave Wang, davewang@wustl.edu.
-
10× concentrated E. coli OP50-1
-
Grown in LB medium at 37°C and 200 to 300 rpm shaking overnight, concentrated by pelleting, and removing supernatant.
-
20°C incubator
-
Nutator (Labquake Shaker Rotisserie, Model 4125110)
-
15- and 50-ml conical tubes
-
Laminar flow hood
-
10-ml repeater pipette with 5-ml tip
-
Fluorescence microscope
-
Glass Pasteur pipette
-
Benchtop centrifuge
-
1.5-ml centrifuge tubes
-
Sterile 1.0-mm diameter silicon carbide beads (BioSpec Products, cat. no. 11079110sc)
-
Bead beater (Scientific Industries Disruptor Genie, Model SI-D236)
-
Dissecting microscope
-
10-ml syringe
-
0.22-µm filter
-
Ice
-
Liquid nitrogen
Obtain a population of developmentally synchronized worms
1.Chunk (i.e., cut pieces of agar containing large numbers of C. elegans) from two 6-cm freshly starved plates of rde-1; jyIs8 worms onto ten 10-cm seeded NGM plates.
2.Incubate at 20°C for 3 days.
3.Bleach gravid adults to release eggs.
4.Incubate embryos in 3 ml M9 buffer at 20°C with rotation on a nutator overnight (18 to 24 hr) to yield a synchronized population of L1 stage animals.
Plate and infect L1s
5.In a 50-ml conical tube, prepare an infection master mix containing L1 worms, 10× E. coli OP50-1, Orsay virus filtrate (OVF), and M9 buffer. Prepare enough infection mix for at least thirty plates (1 ml per plate).
6.In a laminar flow hood, use a repeater pipette to dispense 1 ml of infection mix onto (at least) thirty 10-cm plates. Tilt to spread liquid evenly across the surface of the plate.
7.Allow plates to dry uncovered in laminar flow hood until all liquid disappears.
8.Cover plates and incubate at 20°C until L1s are gravid adults (∼60 hr).
Grind infected worms
9.Confirm infection by fluorescence microscope.
10.Use M9 to collect worms into 15-ml conical tubes, pooling three plates into one tube, as follows:
-
Begin by adding 7 ml of M9 to one plate and tilt to collect worms into solution.
-
Pour liquid from the first plate onto the second plate and tilt to collect worms into solution.
-
Repeat with the third plate.
-
Transfer the worms to a 15-ml conical tube by pouring or by using a glass Pasteur pipette (instead of plastic, because worms adhere less to glass than to plastic).
-
Repeat the process with an additional 7 ml of M9 to collect any remaining worms on the three plates.
11.Wash worms twice with M9.Pellet worms using a benchtop centrifuge 30 s at 1500 × g , room temperature, remove supernatant to ∼2 ml, and resuspend in 10 ml of M9. Repeat steps for the second wash.
12.Pellet worms, resuspend in 1 ml of M9, and transfer to 1.5-ml microcentrifuge tubes.
13.Add a scoop of sterile silicon carbide beads to each 1.5-ml tube.
14.Transfer tubes to the bead beater and shake for ∼30 min at full speed (3000 rpm). Check for homogeneity under a dissecting microscope. Not all worm tissue will be dissolved but ensure that no large chunks remain (Fig. 2A).
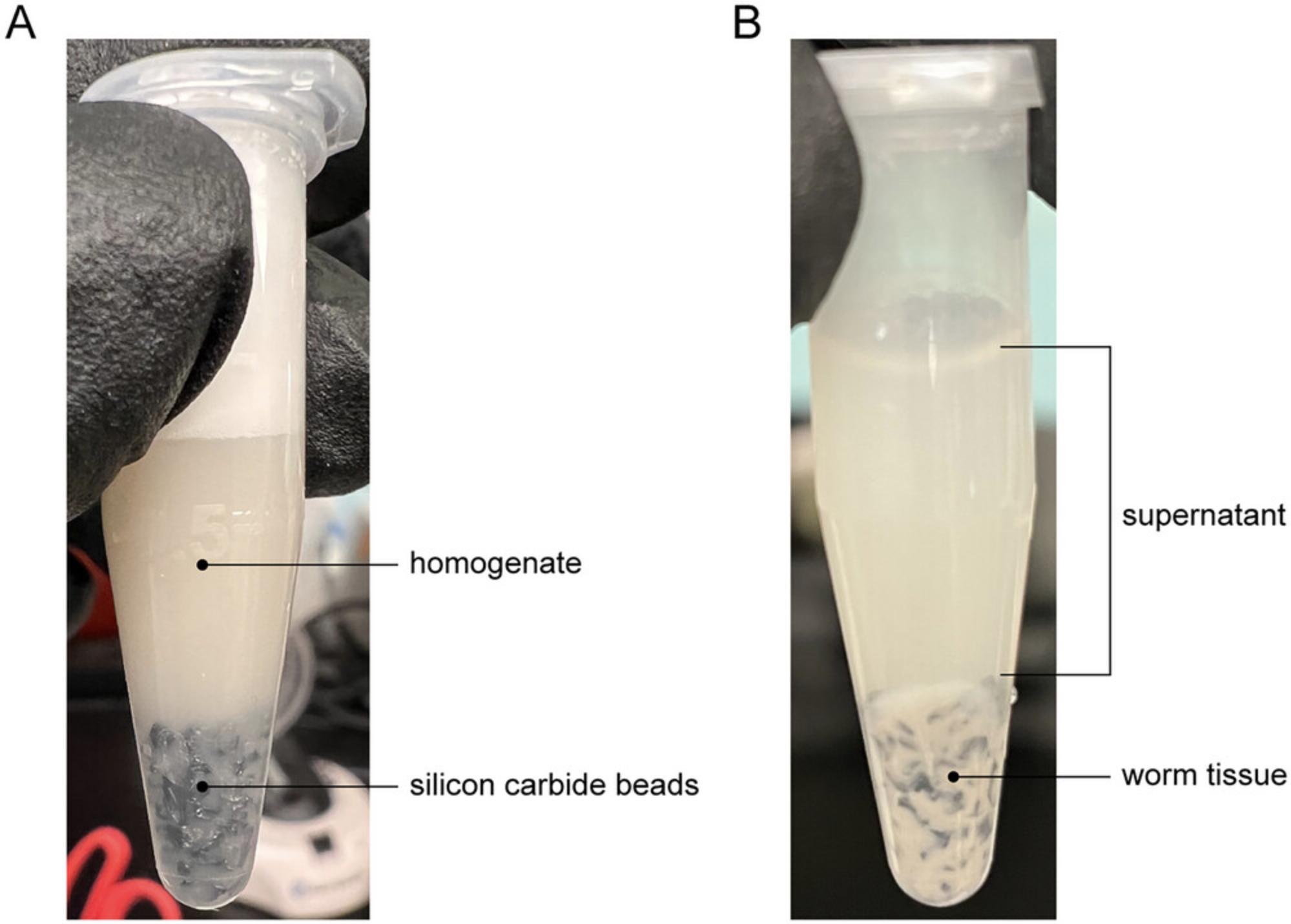
Filter supernatant
15.Spin tubes in benchtop centrifuge 2 min at maximum speed (~21,000 × g), room temperature.
16.Transfer supernatant from 1.5-ml tubes into a 50-ml conical tube (Fig. 2B).
17.Use a 0.22-µm filter and 10-ml syringe to filter-sterilize 10 ml of the supernatant into a fresh 50-ml conical tube on ice.
18.In a sterile environment and on ice, aliquot 100 µl of filtrate into each 1.5-ml tube.
Flash freeze aliquots
19.Flash freeze aliquots in liquid nitrogen.
20.Once frozen, place tubes into a box labeled with the lot number, date of preparation, and initials. Store at −80°C.
Assess infectivity of virus filtrate
Perform infections at the L4 stage and assess infectivity at 24 hr post-inoculation (hpi).
21.Seed 6-well NGM plates with 200 µl of 10× E. coli OP50-1 per well. Incubate at room temperature for 2 days. Store at 4°C until L1s are ready to be plated.
22.Pick 20 to 40 L4 stage worms onto 10-cm NGM plates seeded with E. coli OP50-1.
23.Incubate worms at 20°C for 87 to 90 hr until progeny become gravid adults.
24.Bleach gravid adults to release eggs.
25.Incubate eggs at 20°C with rotation overnight (18 to 24 hr) to yield a synchronized population of L1s.
26.Plate 200 L1s in each well of the seeded 6-well NGM plates. Incubate at 20°C for 40 to 48 hr until worms reach the L4 stage.
27.Set up an infection dosage curve by performing serial 10-fold dilutions of the virus filtrate across seeded 6-well NGM plates. Each well should contain 50 µl of 10× E. coli OP50-1, 3 µl virus filtrate, and 47 µl M9 in a total volume of 100 µl. Set up triplicates for each of the following OVF dilutions: 100, 10-1, 10-2, 10-3, 10-4, and 0.
28.Add 100 µl of infection mix to each well containing L4 animals.
29.Incubate at 20°C for 24 hr.
30.Using a fluorescence microscope, determine the percentage of animals expressing pals-5p ::GFP across the different virus filtrate dilutions.
31.To determine the infectivity of the virus filtrate, proceed to Basic Protocols 3 and 4 to carry out qRT-PCR and fluorescence in situ hybridization (FISH), respectively.
Support Protocol: SYNCHRONIZE C. elegans DEVELOPMENT BY BLEACHING
The steps below describe how to synchronize a population of C. elegans animals at the L1 larval stage. In this protocol, gravid adults are exposed to bleach to dissolve the worm cuticle. By limiting the duration of bleach exposure, this technique results in the release of eggs without killing the embryos, as the eggshells provide protection against bleach. Eggs are then allowed to hatch in minimal media overnight to obtain starved L1 animals. Once exposed to food, L1s resume synchronous growth.
Materials
-
L4 stage worms (see Basic Protocol 1)
-
Standard 10-cm NGM plates seeded with E. coli OP50-1 (Stiernagle, 2006)
-
10× concentrated E. coli OP50-1
-
M9 buffer (see recipe)
-
5.65% to 6% sodium hypochlorite (Fisher Chemical, cat. no. SS290)
-
5 M sodium hydroxide (NaOH) (Fisher Chemical, cat. no. SS256)
-
20°C incubator
-
Glass Pasteur pipette
-
15-ml conical tubes
-
Clinical centrifuge (Thermo Scientific Sorvall ST 8R Centrifuge, cat. no. 75007203)
-
Dissecting microscope
-
Nutator (Labquake Shaker Rotisserie, Model 4125110)
-
Glass slides
NOTE : Bleach loses potency over time due to oxidation. If the bleach is old, worms may need to be exposed to bleach for a greater duration than recommended below in order to dissolve the cuticle.
Bleach gravid adults
1.Pick 20 to 40 L4 stage worms onto standard 10-cm NGM plates seeded with E. coli OP50-1.Supplement plates with 1 ml of 10× E. coli OP50-1.
2.Incubate plates at 20°C for 87 to 90 hr until most of the progeny become gravid adults.
3.Wash gravid adults off the plate with 15 ml of M9 buffer and transfer worms into a 15-ml conical tube using a sterile glass Pasteur pipette.
4.Pellet worms in clinical centrifuge 30 s at 1500 × g , room temperature.
5.Remove supernatant until there is ∼2 ml liquid remaining in the tube.
6.Add 800 µl of 5.65% to 6% sodium hypochlorite and 200 µl of 5 M NaOH to the worm suspension. Shake or vortex vigorously. Observe worms with a dissecting microscope until they break apart and release eggs.
7.Add M9 to a volume of 14 ml. Perform this step quickly.
8.Spin down sample 30 s at 1500 × g , room temperature.
9.Remove supernatant by decanting.
10.Repeat steps 7 to 9 for a total of 5 washes.
11.Resuspend eggs in 3 to 5 ml M9.
12.Incubate eggs at 20°C with rotation using a nutator overnight (18 to 24 hr) to yield a synchronized population of L1s.
13.After 18 to 24 hr, determine the concentration of L1s in M9 by pipetting 5 µl of the L1 suspension onto a glass slide. Count the number of L1s in the drop. Repeat two more times and calculate the total number of L1s per µl. If the L1 density is too low, concentrate L1s by spinning at 30 s at 1500 × g , room temperature. If the density is too high, dilute with M9.
Basic Protocol 2: ORSAY VIRUS INFECTION
Orsay virus infections are performed by feeding C. elegans a mixture of E. coli OP50-1 as a food source together with virus filtrate, which can be prepared by following Basic Protocol 1.While infections can be performed at any developmental stage, the protocol below describes the steps for performing infections at both L1 and L4 stages (Fig. 1B). Advantages of infecting L1s, compared to infecting animals at later stages, are that worms can be used immediately after hatching and can be plated simultaneously with food and virus. Thus, L1 infections do not require pre-seeded plates. An advantage of performing L4 infections is the ability to compare the outcome of viral infection with the outcome of infection by other pathogens, especially as bacterial infections are commonly performed at the L4 stage. Additionally, L4 infections are ideal if downstream analysis includes qRT-PCR, as L4s and adults yield greater amounts of RNA than younger animals. Depending on the experimental setup, infections can be performed using various plate formats. Here, we describe protocols for setting up infections on 6-well, 6-cm, and 10-cm plates.
Materials
-
Unseeded 6-well, 6-cm, or 10-cm NGM plates (Stiernagle, 2006)
-
10× concentrated E. coli OP50-1
-
Standard 10-cm NGM plates seeded with E. coli OP50-1
-
Bleaching solution (see Support Protocol)
-
Orsay virus filtrate (OVF) (see Basic Protocol 1)
-
M9 buffer (see recipe)
-
Laminar flow hood
-
20°C incubator
-
Nutator (Labquake Shaker Rotisserie, Model 4125110) Laminar flow hood
-
15-ml conical tubes
Seed NGM plates with E. coli OP50-1 (L4 infections only)
Seeded plates will be used for performing L4 infections (see steps 11 to 17 in the ‘Perform infection’ section below).
1.Proceed to step 4 for L1 infections or step 2 for L4 infections.
2.If using 6-well or 6-cm plates, add 200 µl of 10× E. coli OP50-1 to plates and incubate at room temperature for 2 days. If using 10-cm plates, add 500 µl of 10× E. coli OP50-1 to plates and incubate at room temperature for 5 days.
3.After incubation, use plates immediately or store at 4°C for up to 1 month.
Obtain developmentally synchronized populations of worms
4.Pick 20 to 40 L4 stage worms onto 10-cm NGM plates seeded with E. coli OP50-1.
5.Incubate worms at 20°C for 87 to 90 hr until progeny become gravid adults.
6.Bleach gravid adults to release eggs.
7.Incubate eggs at 20°C with rotation using a nutator overnight (18 to 24 hr) to yield a synchronized population of L1s.
Perform infection
Bring 10× E. coli OP50-1 to room temperature prior to making up master mix. The working dilution for Orsay virus filtrate will depend on the preparation described in Basic Protocol 1.
8.Proceed to step 9 for L1 infections or step 11 for L4 infections.
9.For L1 infections, make up an infection master mix containing L1 worms, 10× E. coli OP50-1, Orsay virus filtrate, and sterile M9 buffer in a 15-ml conical tube. Additionally, make up an uninfected control master mix by replacing Orsay virus filtrate with sterile M9.
10.Add 100 µl per well (6-well plate), 300 µl (6-cm plate), or 900 µl (10-cm plate) of infection mix to each plate and tilt to spread liquid evenly across the surface of the plate. Proceed to step 15.
11.For L4 infections, plate the following number of worms onto each pre-seeded plate: 200 L1s per well (6-well plates), 700 L1s (6-cm plates), or 2000 L1s (10-cm plates). See steps 2 to 3 for seeding plates.
12.Incubate plates at 20°C for 40 to 48 hr until worms reach the L4 stage.
13.In a 15-ml conical tube, make up an infection master mix containing 10× E. coli OP50-1, Orsay virus filtrate, and sterile M9.Additionally, make up an uninfected control master mix by replacing Orsay virus filtrate with sterile M9.
14.Add 100 µl per well (6-well plate), 300 µl (6-cm plate), or 900 µl (10-cm plate) of infection mix to each plate and tilt to spread liquid evenly across the surface of the plate.
15.Allow plates to dry uncovered in laminar flow hood until all liquid disappears.
16.Incubate plates at 20°C for 18 hr (L1 infections) or 24 hr (L4 infections).
17.Assess infection outcomes (see Basic Protocols 3 and 4).
Basic Protocol 3: QUANTIFICATION OF ORSAY VIRUS RNA1/RNA2 TRANSCRIPT LEVELS BY qRT-PCR
The outcome of viral infection can be quantified using several different approaches, including qRT-PCR (Fig. 1C) and/or fluorescence in situ hybridization (FISH) (Fig. 1D). Choosing which method(s) to use for analysis depends on the expected magnitude of the effect, although this magnitude may not be known prior to performing the experiment. In Basic Protocol 3, we describe how to measure Orsay virus RNA1 and RNA2 transcript levels with qRT-PCR. Analysis by qRT-PCR is a direct measurement of viral load and provides useful information when large differences are expected between samples.
Materials
- Infected C. elegans in 10-cm plates (from Basic Protocol 2)
- M9 buffer (see recipe)
- RNase-free surface decontaminant (Apex Bioresearch Products, cat. no. 10-228)
- TRI reagent (MRC, cat. no. TR118)
- BCP phase separation reagent (MRC, cat. no. BP151)
- Isopropanol (Sigma, cat. no. I9516-500ML)
- 100% ethanol (Volu-Sol, cat. no. VAE-016)
- H2O, HyPure molecular biology grade (GE Life Science, cat. no. SH30528.03)
- iScript cDNA synthesis kit (Bio-Rad, cat. no. 1708890)
- iQ SYBR Green Supermix (Bio-Rad, cat. no. 1708880)
- qPCR primers (see Table 1)
Target | Primer name | Sequence | Source |
---|---|---|---|
RNA1 | GW194 | ACCTCACAACTGCCATCTACA | Félix et al. (2011) |
GW195 | GACGCTTCCAAGATTGGTATTGGT | ||
RNA2 | GW303 | CCGGCGACAATGTGTACCA | Jiang et al. (2014) |
GW304 | CCAGCCCTCCGTTGACAA |
- Glass Pasteur pipette and bulb
- Sterile 15-ml conical tubes
- Clinical centrifuge (Thermo Scientific Sorvall ST 8R Centrifuge, cat. no. 75007203)
- Filter pipette tips
- Sterile 1.5-ml microcentrifuge tubes
- Mini centrifuge
- NanoDrop spectrophotometer
- 0.2-ml PCR tubes
- PCR thermocycler
- 96-well qPCR plate
- Microseal ‘B’ PCR plate sealing film (Bio-Rad, cat. no. MSB1001)
- Real-time PCR system
CAUTION : TRI reagent is highly toxic. Avoid exposure to the skin and airways.
CAUTION : BCP phase separation reagent is a flammable liquid and is toxic if ingested or inhaled. Use in a well-ventilated area and keep away from open flames.
Extract RNA
This protocol is optimized for measuring mRNA transcript levels in L4s and adults. 1000 L4 animals yield ∼10 µg total RNA. Always use filter pipet tips when performing RNA extractions. Keep RNA tubes and reagents separate from other reagents. RNA is most vulnerable once it has been extracted from cells.
1.Add 15 ml M9 buffer to a 10-cm plate containing infected worms (from Basic Protocol 2).
2.Use a glass Pasteur pipette to transfer worms to a 15-ml conical tube.
3.Pellet worms by centrifuging 30 s at 1500 × g , room temperature, and aspirate supernatant to 100 µl.
4.Add 15 ml M9, pellet worms 30 s at 1500 × g , room temperature, and aspirate supernatant to 100 µl.
5.Wipe down work area, reagents, and equipment with RNase-free surface decontaminant.
6.Using filter pipet tips, add 1 ml TRI reagent to 100 µl of packed worms and homogenize by slowly pipetting up and down 10 times. Transfer mixture to a 1.5-ml microcentrifuge tube.
7.Store homogenate at room temperature for 10 to 30 min.
8.Add 100 µl BCP to each sample, press down against the lid of the tube to prevent leaking, and shake vigorously for 15 s.
9.Store mixture at room temperature for 2 to 15 min.
10.Spin 15 min at 12,000 × g , 4°C.
11.Transfer the aqueous phase (clear layer) to a new tube without disturbing the interphase (cloudy layer), which contains genomic DNA.
12.Add 500 µl isopropanol, mix by inverting tubes, and store at room temperature for 5 to 10 min to precipitate the RNA.
13.Spin 15 min at 12,000 × g , 4°C. Remove the supernatant by pipetting.
14.Add 1 ml of 75% ethanol to wash the RNA pellet.
15.Centrifuge 5 min at 7500 × g , 4°C.
16.Remove the ethanol first with a large-bore pipet tip (i.e., 1000 µl). Perform a quick spin down of tubes using a mini centrifuge at room temperature and remove any remaining ethanol with a smaller pipet tip (i.e., 200 µl or 10 µl), making sure not to disturb the pellet. A white pellet should be visible if RNA yield is >10 µg.
17.Leave tubes uncovered and monitor until the pellet becomes translucent, which indicates that all ethanol has evaporated.
18.As soon as the pellet becomes translucent, resuspend in 20 to 30 µl nuclease-free water and incubate at room temperature for 10 min to dissolve pellet. Store RNA samples at −80°C.
Synthesize cDNA
cDNA synthesis is performed with the iScript cDNA synthesis kit.
19.Measure the concentration of RNA on a NanoDrop.
20.Make up a master mix containing iScript reverse transcriptase, 1× iScript buffer, and nuclease-free water.
21.In a 0.2-ml PCR tube, add 5 µl iScript master mix, 500 ng RNA, and nuclease-free water to a total volume of 10 µl.
22.Run the reaction protocol on a thermocycler following the kit instructions.
Run qRT-PCR
23.Make up a master mix containing iQ SYBR Supermix and primers targeting Orsay virus RNA1 and/or RNA2 (Table 1). Make up an additional master mix using primers targeting the housekeeping gene. Include a minimum of duplicate reactions per sample.
24.In a 96-well qPCR plate, pipet 10 µl of reaction mix into each well. Seal plate with film.
25.Run the reaction protocol on a real-time PCR system.
Basic Protocol 4: QUANTIFICATION OF INFECTION RATE AND FISH FLUORESCENCE INTENSITY
This protocol describes how to measure the percentage of infected worms within a population by FISH staining (Part 1) and how to quantify FISH fluorescence intensity as a proxy for pathogen load (Part 2). By assessing individuals within a population, FISH analyses are better able to distinguish small differences in viral resistance. Analysis by qRT-PCR analysis can lack sensitivity in discriminating modest differences, as qRT-PCR measures the viral load of an entire sample without accounting for individual heterogeneity within the sample. For example, several heavily infected worms within a population can skew the viral load measurement, thus obscuring the effects of uninfected worms, which is more clearly detected by FISH.
Materials
- C. elegans strains
- Orsay virus RNA1 and RNA2 FISH probes (Biosearch Technologies) (Table 2)
- 16% paraformaldehyde (Electron Microscopy Sciences, cat. no. 15710)
- VECTASHIELD antifade mounting medium (Vector Laboratories, cat. no. H-1000-10), optional
- Agarose
- FISH hybridization and wash buffers (see recipes)
Target gene | Fluorophore | Sequence | Source |
---|---|---|---|
RNA1 | Cal Fluor Red 610 (CF610) | GACATATGTGATGCCGAGAC | Biosearch Technologies |
Quasar 670 (Q670) | |||
RNA2 | Cal Fluor Red 610 (CF610) | GTAGTGTCATTGTAGGCAGC | |
Quasar 670 (Q670) |
- Glass microscope slides
- 22-mm × 22-mm cover slip
- Fluorescence microscope
- Glass Pasteur pipette
- Fiji (see Internet Resources)
CAUTION : Paraformaldehyde is a carcinogenic substance that becomes toxic when exposed to the skin and airways. Any steps involving paraformaldehyde should be performed in a fume hood or well-ventilated area.
Hybridize FISH probes
1.Perform FISH staining of C. elegans following the protocol detailed in Rivera et al. (2022). Use probes targeting Orsay virus RNA1 and RNA2 genomic segments (Table 2).
2.Proceed to Part 1 to measure the infection rate and Part 2 to quantify FISH fluorescence intensity.
Part 1: Quantification of the percentage of worms with any evidence of infection by FISH staining
3.Add ∼5 µl of sample onto a glass microscope slide and place a 22-mm × 22-mm cover slip on top.
4.Under a fluorescence microscope, manually score animals and record the percentage of animals that show positive FISH staining. As a control for positive staining, it is recommended to include a condition in which highly susceptible animals, e.g., rde-1 mutants, are infected with virus. As a negative control, FISH staining should also be performed on uninfected animals.
Part 2: Quantification of FISH fluorescence intensity
5.Make a 5% agarose pad on a glass microscope slide as follows:
-
Dissolve agarose in water to make a 5% agarose solution.
-
Using a glass Pasteur pipette, add a drop of the agarose solution to a glass microscope slide.
-
Immediately place another glass slide on top of the drop to create a circular pad.
-
Once the agarose has dried, carefully separate both slides while leaving the agarose intact on one of the slides.
6.Add ∼5 µl of sample onto the agarose pad and place a 22-mm × 22-mm cover slip on top of the sample.
7.Acquire images on a fluorescence microscope.
8.Using Fiji software, quantify background-corrected mean fluorescence intensity (MFI) as follows:
-
Open images for all channels by dragging and dropping image files into Fiji.
-
Merge transmitted light (TL) and fluorescent channels by selecting “Image” > “Color” > “Merge Channels…”.
-
To set measurement parameters, select “Analyze” > “Set Measurements…”.
-
Select “Mean gray value” > “OK”.
-
Within the image stack, switch over to the fluorescence channel corresponding to the fluorescent probe used for FISH staining.
-
Use the polygon selection tool to trace around the border of the worm.
Only trace worms that are fully visible and remained motionless during imaging. Do not trace overlapping worms or those that touch the border of the well.
-
Select “Analyze” > “Measure” to record the mean gray value, or mean fluorescence intensity (MFI), of the worm.
-
To measure background fluorescence, left click and drag the polygon shape to an empty space in the well next to the worm. Select “Analyze” > “Measure”.
-
Calculate background-corrected fluorescence by subtracting the background fluorescence from the worm fluorescence using the equation below.$$\begin{equation*} {\rm{MF}}{{{\rm{I}}}{{\rm{corrected}}}} = {\rm{MF}}{{{\rm{I}}}{{\rm{worm}}}}-{\rm{MF}}{{{\rm{I}}}_{{\rm{background}}}} \end{equation*}$$
Basic Protocol 5: IMMUNOFLUORESCENT LABELING OF dsRNA IN VIRUS-INFECTED INTESTINAL TISSUE
Performing immunohistochemistry in adult C. elegans intestinal cells is not straightforward due to challenges in efficiently delivering antibody across the worm cuticle and into cells, in addition to the high levels of autofluorescence in adult intestinal cells. Here, we describe how to dissect out the intestine in order to provide increased antibody permeability and perform immunofluorescence in the intestinal tissue of adult C. elegans using the J2 antibody, which targets dsRNA. In addition, we describe how to combine dsRNA immunofluorescence with FISH staining to enable visualization of dsRNA in virus-infected cells (Fig. 1E).
Materials
-
C. elegans strains
-
M9 buffer (see recipe)
-
100 µM levamisole (MP Biomedicals, cat. no. 155228)
-
16% paraformaldehyde (Electron Microscopy Sciences, cat. no. 15710)
-
10× PBS (Current Protocols, 2006)
-
Tween 20 (Thermo Scientific, cat. no. AC233362500)
-
Blocking solution (see recipe)
-
Mouse anti-dsRNA antibody clone rJ2 (Sigma, cat. no. MABE1134-25UL)
-
Goat anti-mouse IgG(H+L) cross-adsorbed secondary antibody, Alexa Fluor 488 (Invitrogen, cat. no. A-11001)
-
Agarose (Sigma-Aldrich, cat. no. A9539)
-
15-ml conical tube
-
Benchtop centrifuge
-
Glass microscope slide
-
25G needle
-
1.5-ml microcentrifuge tubes
-
Glass Pasteur pipette
-
Heat block, 47°C
-
22-mm × 22-mm cover slip
-
Confocal microscope
CAUTION : Paraformaldehyde is a carcinogenic substance that becomes toxic when exposed to the skin and airways. Any steps involving paraformaldehyde should be performed in a fume hood or well-ventilated area.
CAUTION : The blocking solution used in this protocol contains sodium azide, which is a hazardous compound that forms a toxic gas when mixed with water or acid. Work in a fume hood when handling sodium azide.
Infect worms with virus
This protocol is optimized for staining intestines from adult animals.
1.Perform infections at the L4 stage for 24 hr following steps outlined in Basic Protocol 2.
Remove intestines by dissection
2.For each treatment condition, wash worms off of plates with 15 ml M9 and transfer to a 15-ml conical tube.
3.Pellet worms by centrifuging 1 min at 2000 × g , room temperature, and then aspirate supernatant.
4.Wash samples by adding 15 ml M9 to each tube. Pellet worms as in step 3 and aspirate supernatant. Repeat wash if supernatant is cloudy.
5.Pipet 50 µl worms and 5 µl of 100µM levamisole onto a microscope slide.
6.Dissect out 100 to 200 intestines using a 25G needle (Fig. 3). Holding the needle at an angle, use the sharp edge to cut off the head and/or tail of each worm. The internal pressure of the worm will cause the intestine and gonad to ooze out.
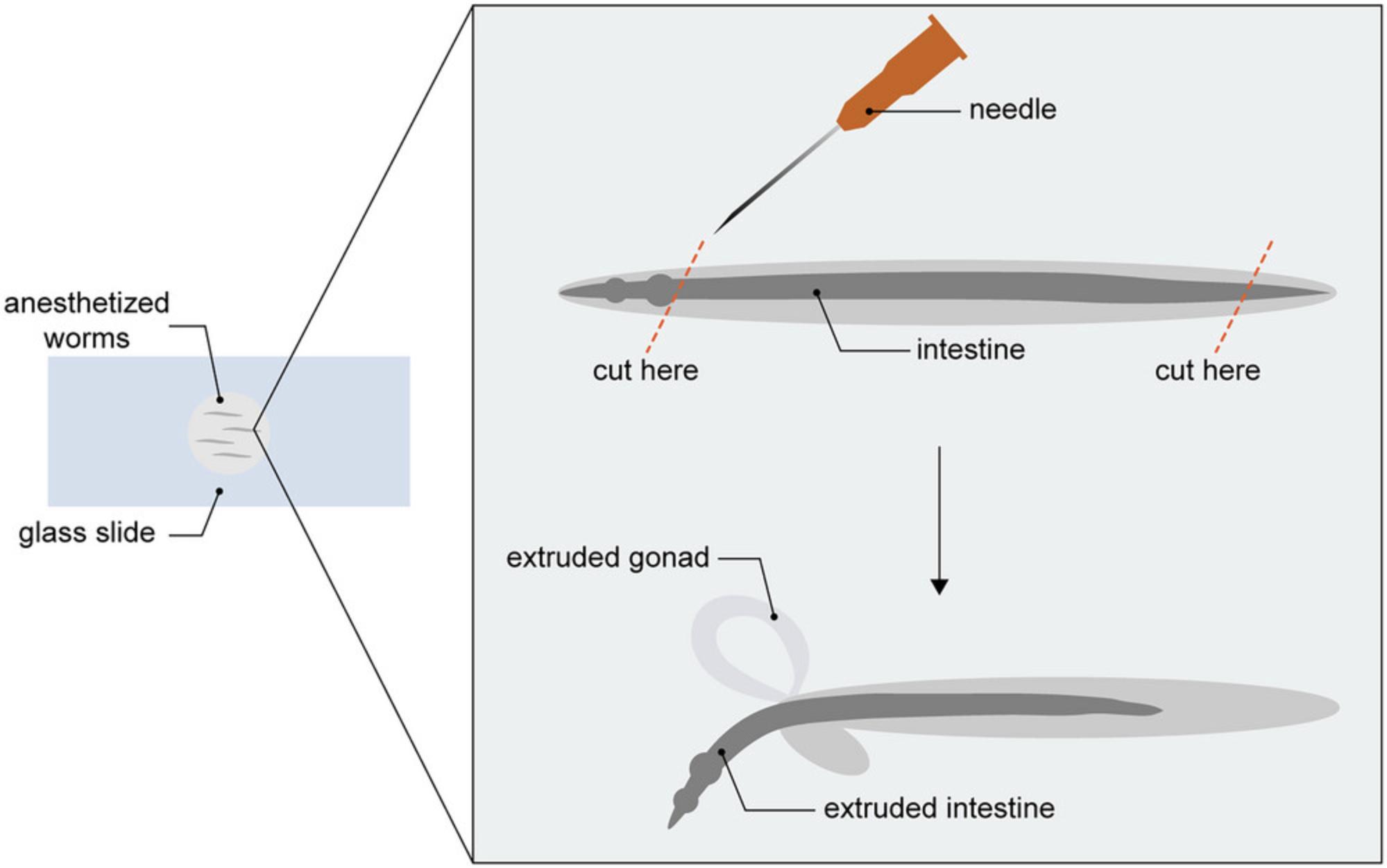
7.Transfer dissected intestines to 1.5-ml microcentrifuge tubes using a glass Pasteur pipette.
8.Centrifuge tubes 1 min at 2000 × g , room temperature, and carefully aspirate supernatant without disturbing dissected intestines. Resuspend in a total volume of 100 µl M9.
Fix samples and stain with FISH probes
9.Add 33 µl of 16% PFA to 100 µl of sample to achieve a final concentration of 4% PFA. Incubate at room temperature for 15 min.
10.Wash samples twice with 1× PBS + 0.1% Tween 20.For each wash, add 1 ml of 1× PBS + 0.1% Tween 20, centrifuge tubes 1 min at 2000 × g , room temperature, and remove supernatant down to ~100 µl.
11.Perform FISH staining as described in Rivera et al. (2022).
Add primary and secondary antibodies
12.Add 200 µl blocking solution to each sample and incubate overnight at 4°C.
13.Centrifuge tubes 1 min at 2000 × g , room temperature, and aspirate supernatant.
14.Add mouse anti-dsRNA J2 antibody and incubate at room temperature for 1 to 2 hr.
15.Wash samples 4 times with 1× PBS + 0.1% Tween 20. For each wash, add 200 µl of 1× PBS + 0.1% Tween 20, centrifuge tubes 1 min at 2000 × g , room temperature, and remove supernatant down to ∼100 µl.
16.Add 4 to 10 µg/ml goat anti-mouse IgG(H+L) Alexa Fluor 488 secondary antibody and incubate in the dark at room temperature for 1 to 2 hr.
17.Wash samples 5 times with 200 µl 1× PBS + 0.1% Tween 20, performing centrifugation as in step 15.
18.Add 100 µl of 1× PBS + 0.1% Tween 20 and store samples at 4°C.
Acquire confocal images
19.Make a 5% agarose pad on a glass microscope slides as follows:
-
Dissolve agarose in water to make a 5% agarose solution.
-
Using a glass Pasteur pipette, add a drop of the agarose solution to a glass microscope slide.
-
Immediately place another glass slide on top of the drop to create a circular pad.
-
Once the agarose has dried, carefully separate both slides while leaving the agarose intact on one of the slides.
20.Pipet ∼5 µl of sample onto the agarose pad and place a cover slip on top of the sample.
21.Acquire images on a confocal microscope.
REAGENTS AND SOLUTIONS
Blocking solution
- 100 ml of 1× PBS (Current Protocols, 2006)
- 0.5 ml of 0.5% Tween 20 (Thermo Scientific, cat. no. AC233362500)
- 5 g of bovine serum albumin (BSA) (5%) (Sigma-Aldrich, cat. no. A3294)
- 0.05 g NaN3 (0.05%) (Sigma-Aldrich, cat. no. 71289)
- Filter sterilize using a 0.22-µm filter
- Store up to 6 months at 4°C
FISH hybridization buffer
- 180 µl of 5 M NaCl (Current Protocols, 2006)
- 20 µl of 1 M Tris pH 7.5 (Current Protocols, 2006)
- 800 µl ddH2O
- 2 µl of 10% sodium dodecyl sulfate (SDS) (Current Protocols, 2006)
- Prepare fresh for each experiment otherwise precipitation will occur
This recipe makes 1 ml of buffer and 200 µl buffer is needed per sample. Scale up accordingly.
FISH wash buffer
- 180 µl of 5 M NaCl (Current Protocols, 2006)
- 20 µl of 1 M Tris pH 7.5 (Current Protocols, 2006)
- 10 µl of 0.5 M EDTA (Current Protocols, 2006)
- 790 µl ddH2O
- 2 µl of 10% SDS (Current Protocols, 2006)
- Prepare fresh for each experiment otherwise precipitation will occur
This recipe makes 1 ml of buffer and 200 µl buffer is needed per sample. Scale up accordingly.
M9 buffer
- For 1 L:
- 5.8 g Na2HPO4 (Current Protocols, 2006)
- 3.0 g KH2PO4 (Current Protocols, 2006)
- 0.5 g NaCl (Current Protocols, 2006)
- 1.0 g NH4Cl
- 1 L ddH2O
- Sterilize by autoclaving
- Store up to 9 months at room temperature
COMMENTARY
Background Information
In non-mammalian hosts, the study of antiviral defense has led to landmark discoveries, including CRISPR and RNA interference (RNAi). Until the discovery of the Orsay virus in 2011 (Félix et al., 2011), the absence of a known viral pathogen capable of infecting and undergoing its entire life cycle inside C. elegans presented a limitation to the use of C. elegans as a model organism for studying host-virus interactions. In early work, studies of antiviral defense were carried out using genetic (Lu et al., 2005), ex vivo (Schott et al., 2005; Wilkins et al., 2005), or artificial approaches (Liu et al., 2006) to model viral infection in C. elegans. The isolation of the Orsay virus and the development of standardized protocols, including those presented above, expands the potential of C. elegans as an experimental system for studying host-virus interactions.
In addition to advancing what is known about antiviral RNAi, which has been extensively studied, the investigation of Orsay virus infection within the last decade has revealed additional genes and pathways that participate in C. elegans antiviral defense. For example, mutagenesis and RNAi screens have uncovered host factors (sid-3, viro-2, nck-1 , and hipr-1) that regulate the outcome of viral infection at early, prereplication life stages of the virus (Jiang et al., 2017, 2020). Follow-up studies have demonstrated that these proteins share conserved function with their mammalian homologs (Jiang et al., 2019, 2020). Recent studies have also implicated a role for structural proteins (i.e., actin, collagen) and the endoplasmic reticulum in mediating antiviral defense (Efstathiou et al., 2022; Zhou et al., 2024). Curiously, several of the collagen components identified as antiviral factors in C. elegans are homologs of human proteins involved in innate immune responses (Zhou et al., 2024). These findings lend support to the potential of the C. elegans -virus model for discovering novel antiviral factors that are conserved in mammals. Future work can clarify the functional role of these proteins in C. elegans antiviral defense.
The study of viral infection in C. elegans has facilitated the discovery of novel host immune responses, such as the Intracellular Pathogen Response (IPR), which is induced by infection with intracellular pathogens, including the Orsay virus. While the IPR is distinct from the well-studied antiviral RNAi response, the regulation of both the IPR and RNAi during infection involves DRH-1, a mammalian RIG-I-like receptor (RLR) homolog. In mammals, RLRs function as cytosolic RNA sensors that detect viral infection and promote signaling to induce type I interferons. RLR-mediated regulation is one of several similarities shared by the type I interferon response and the IPR (Lažetić et al., 2023). However, downstream components of the canonical RLR pathway are absent in C. elegans , presenting an opportunity for the discovery of non-canonical signaling factors that regulate transcriptional responses to viral infection. Taken together, these findings highlight exciting avenues for further exploration into the evolution of host immune responses and the fundamental principles of antiviral immunity.
The techniques presented above offer several advantages for investigating antiviral immunity. One of the central advantages includes the use of microscopy-based tools to visualize viral infection in the context of an entire organism. Furthermore, the rapid life cycle of C. elegans , along with the rapid spread of Orsay virus infection, enables experiments to be performed on a relatively short timescale. Further streamlining infection workflows, Orsay virus filtrate can be generated and stored in large batches, and infection can be easily achieved through feeding. Despite the strengths of this model system, we acknowledge that there are limitations to the protocols described in this article. Namely, the inability to label Orsay virus with a genetically encoded fluorescent tag remains a major obstacle in understanding viral dynamics, which is challenging to assess by FISH due to the need for sample fixation. Another limitation is the lack of a tissue culture system that supports amplification and characterization of the Orsay virus.
While immunofluorescence methods for C. elegans have been previously described, our workflow (Basic Protocol 5) enables simultaneous staining of dsRNA and viral RNA in the intestine of adult worms. Earlier work pioneered the use of immunofluorescence to target viral proteins in the tissue of intact animals (Franz et al., 2014). This study was the first to report the localization of viral proteins in a natural C. elegans infection model. Findings from this study defined the tissue tropism of the Orsay virus as well as the subcellular localization of viral proteins within an intact animal. Viral proteins were visualized in larvae, as the authors noted that performing immunofluorescence on adult animals was challenging due to considerable levels of non-specific staining. Here, we optimize an immunofluorescence assay for staining dsRNA in adult intestinal cells with high specificity, in combination with FISH staining for viral RNA. A limitation of Basic Protocol 5 is the need to dissect intestines for performing immunofluorescence, which increases the duration of the protocol and can introduce unintended effects caused by manipulation of the tissue. While it can be labor-intensive, dissection increases the efficiency of antibody uptake into intestinal cells, allowing for more robust staining. Applications of the immunofluorescence workflow described in Basic Protocol 5 are not limited to dsRNA staining, as this workflow can be adapted for use with an antibody against any desired target.
Critical parameters
For Orsay virus infections, several experimental parameters might influence the infection outcome, including lot-to-lot variation of virus filtrate preparations, handling of virus filtrate, feeding rate, bacterial food source, and overbleaching embryos. Prior to setting up an experiment, the optimal dose of virus filtrate should be empirically determined. Experiments should be performed using the same lot of virus filtrate. Of note, virus filtrate loses infectivity with each freeze-thaw cycle. To maintain infectivity of Orsay virus filtrate preparations, the filtrate should be divided into small aliquots for a single use (Basic Protocol 1). Discard the aliquot once it has been thawed. As Orsay virus infection is established through feeding, any changes to the feeding rate of the C. elegans strain or treatment group may impact the rate of inoculation. Similarly, changes to bacterial food sources, which can influence the feeding rate, can also affect the infection rate. Other factors that can influence the outcome of infection are overbleaching gravid adults and/or incomplete removal of the bleaching solution once eggs are released. Prolonged exposure to bleach can result in the death of the released embryos. Similarly, residual bleach left overnight can kill hatched larvae or cause asynchronous development, introducing variability to infection assays.
Troubleshooting
The tables below list potential solutions for problems that may occur when performing RNA extraction (Table 3), FISH staining (Table 4), and dsRNA immunofluorescence (Table 5).
Problem | Possible cause | Solution |
---|---|---|
Low RNA yield | Insufficient starting sample | Collect RNA from at least 1000 L4s or adults per sample for optimal yields; adults yield more RNA compared to L4s |
Incomplete resolubilization of RNA pellet | After obtaining RNA pellet, perform 10 min incubation at a higher temperature (i.e., ∼50°C) and store at −80°C overnight to promote resolubilization |
Problem | Possible cause | Solution |
---|---|---|
High background fluorescence | Insufficient removal of FISH probe | Increase duration and/or number of washes following hybridization step (e.g., wash 3× with 500 µl wash buffer) |
Problem | Possible cause | Solution |
---|---|---|
Little to no sample remaining at the end of protocol | Loss of sample during wash steps | Increase centrifugation time, ensure pellet is visible after each wash |
Increase sample size (i.e., number of dissected intestines) | ||
High background fluorescence | Antibody concentrations too high | Set up antibody titration to determine concentration that yields optimal signal-to-noise ratio |
Antibody incubation time too long | Decrease incubation time (i.e., 1 hr at room temperature) | |
Insufficient blocking | Prepare fresh blocking buffer | |
Insufficient removal of unbound secondary antibody | Increase number of washes following secondary antibody incubation |
Understanding Results
For each virus filtrate preparation (Basic Protocol 1), a dose-response curve should be performed to determine the optimal dose of virus filtrate for downstream experiments (Fig. 4A). The magnitude of the immune response, as measured by pals-5p ::GFP, is dependent on the starting concentration of virus. An optimal dynamic range for infection assays (Basic Protocol 2) can be achieved by determining a working dilution of virus filtrate that results in ∼50% pals-5p ::GFP activation in a wild-type population. For example, a dilution of 0.1 results in ∼50% wild-type pals-5p ::GFP induction in the virus filtration preparation shown in Figure 4A. At this dilution, the sensitivity of the assay is sufficient to detect differences between the percentage of pals-5p ::GFP+ animals when comparing wild-type (jyIs8) and susceptible (rde-1; jyIs8) mutants. When animals are treated with a higher concentration of virus, the difference in pals-5p ::GFP activation between wild-type and susceptible animals is no longer detected (Fig. 4A).
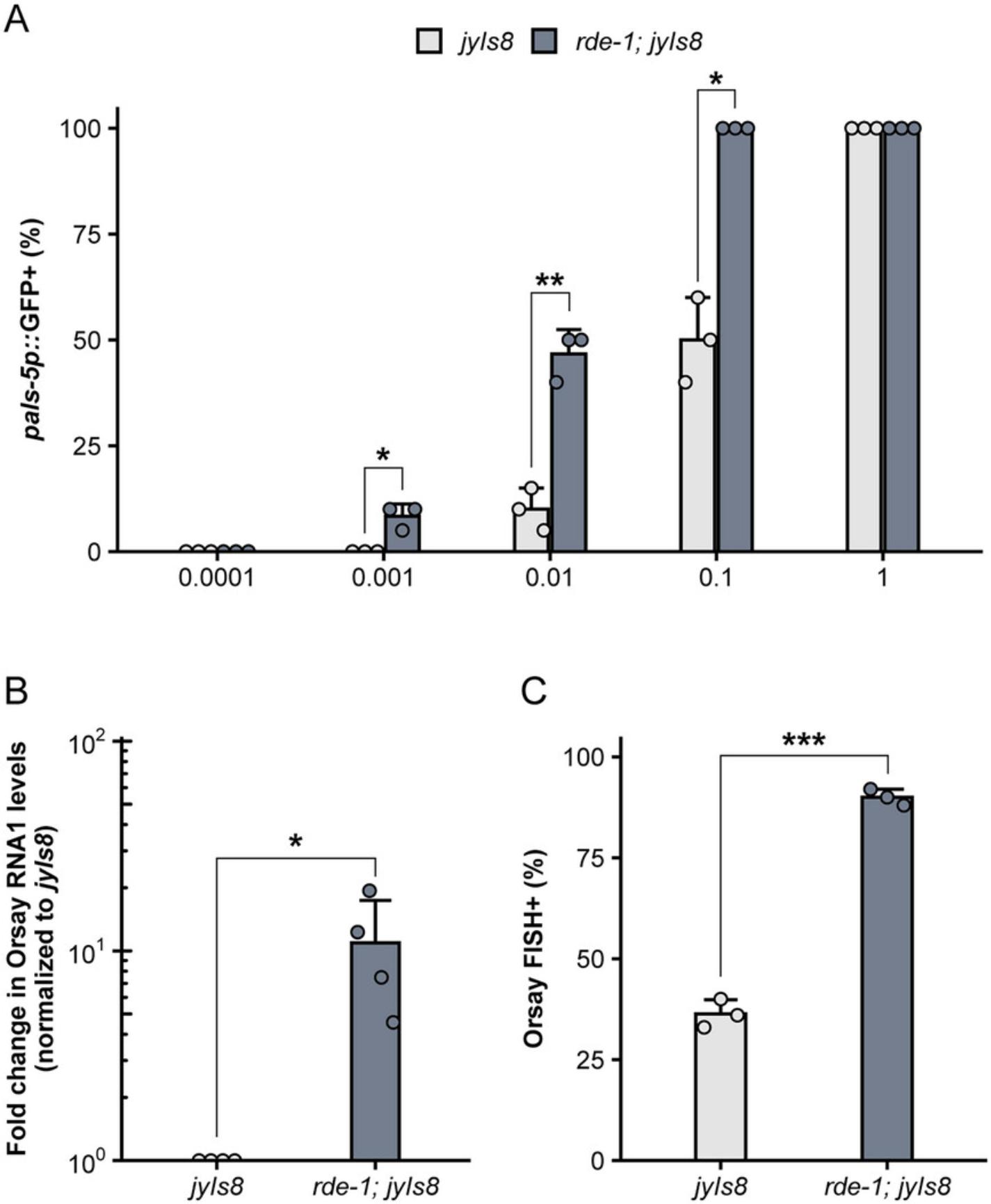
Differences in viral load and infection rate can be quantified by qRT-PCR (Basic Protocol 3) and FISH staining (Basic Protocol 4). Consistent with increased pals-5p ::GFP activation, the level of Orsay virus RNA1 transcripts is elevated in rde-1 mutants compared to wild-type animals (Fig. 4B). Furthermore, the percentage of animals exhibiting Orsay FISH staining is also significantly increased in rde-1 mutants relative to wild-type animals (Fig. 4C). In addition to measuring infection rate within a population, FISH fluorescence can also be used as a readout for infection levels within individual animals. Previous work has demonstrated that pals-22 mutants exhibit a significant decrease in the infection rate, or percentage of FISH+ animals, relative to wild-type animals (Fig. 5A) (Reddy et al., 2019). Here, we demonstrate how mean FISH fluorescence can also be used to quantify the increased resistance in pals-22 mutants (Fig. 5B) and provide a distribution of fluorescence levels within a population.
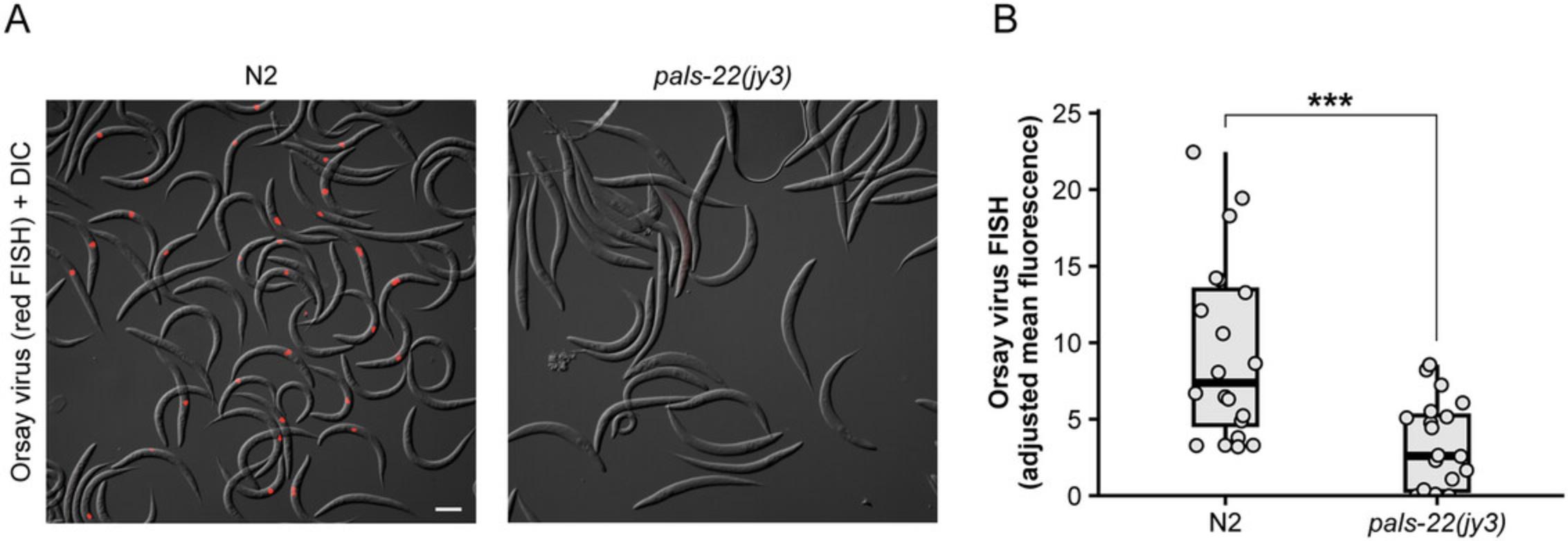
In combination with FISH, immunohistochemistry enables the visualization of both viral RNA and dsRNA at subcellular resolution (Basic Protocol 5). Infected intestinal tissue exhibits bright dsRNA puncta, which are not present in uninfected intestinal cells (Fig. 6) (Batachari et al., 2024). Distributed throughout the cytosol, dsRNA foci are ubiquitous and form ring-like structures. In infected animals, dsRNA is predominantly observed in viral FISH+ cells, whereas neighboring FISH- cells exhibit minimal or no dsRNA fluorescence (Fig. 6), supporting a model in which dsRNA is a product of viral replication. Collectively, Basic Protocols 3 to 5 provide both quantitative and spatial approaches to assessing the outcome of Orsay virus infection.
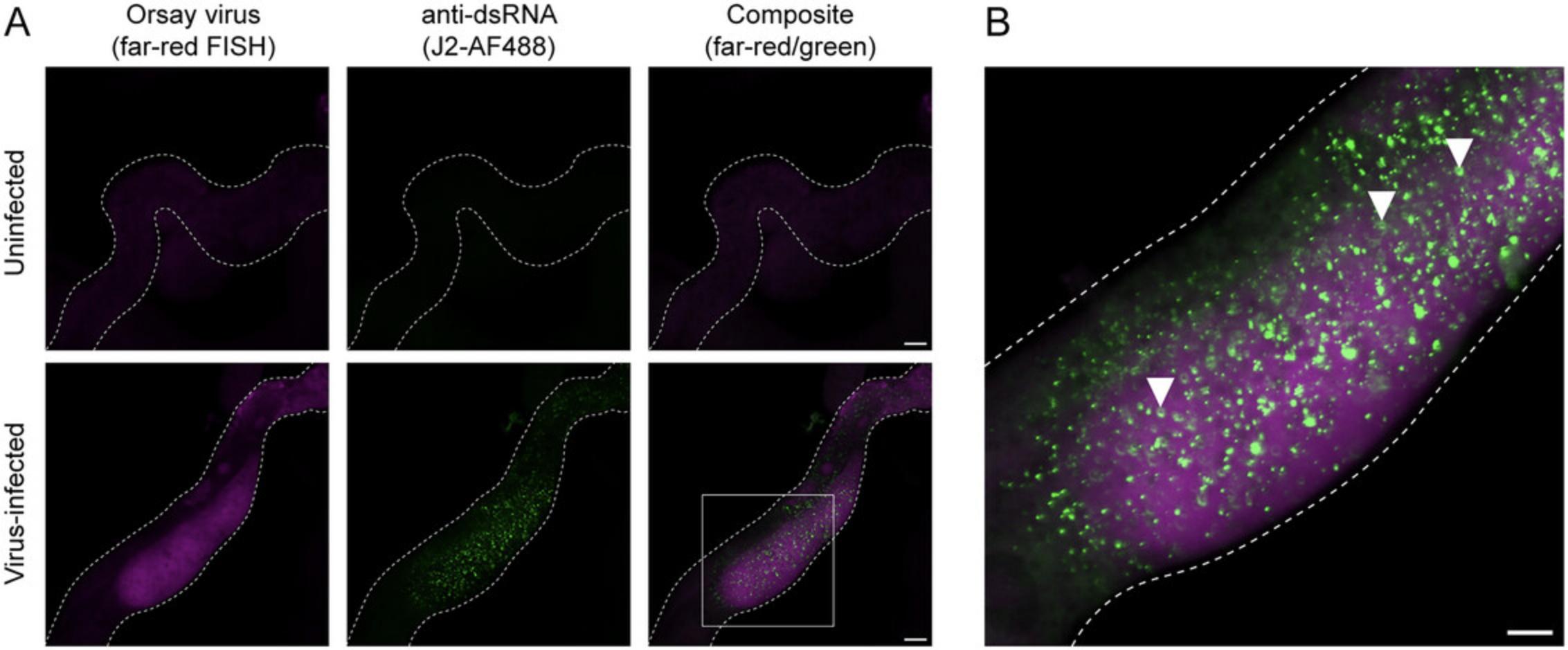
Time Considerations
The preparation of Orsay virus filtrate (Basic Protocol 1) can be completed in 8 days. Assessing the infectivity of the filtrate may take up to an additional 2 weeks depending on which assays are performed. Performing Orsay virus infections (Basic Protocol 2) may take anywhere from 1 to 2 weeks depending on whether infections are performed at the L1 or L4 larval stage. If combining infections with RNAi, the duration of the assay depends on whether RNAi is performed for one or more generations prior to infections. Time estimates should also account for the growth rate of the C. elegans strain used in the experiment. Assays for measuring viral RNA1 and RNA2 transcript levels by qRT-PCR (Basic Protocol 3), FISH fluorescence intensity (Basic Protocol 4), and infection rate (Basic Protocol 4) can be completed in 2 days. Combining FISH staining with dsRNA immunofluorescence can take 2 to 3 days (Basic Protocol 5).
Acknowledgments
The authors thank Brenda Bass, Marie-Anne Félix, Aundrea Koger, Katie Li, Deevya Raman, Supraja Ranganthan, Max Strul, and David Wang for providing helpful feedback on this manuscript. This work was supported by NIH under R01 AG052622, GM114139, AI176639 and by NSF 2301657 to E.R.T. N.W. is supported by Deutsche Forschungsgemeinschaft (DFG, German Research Foundation) under Project 510766555.
Author Contributions
Lakshmi E. Batachari : Conceptualization; data curation; formal analysis; investigation; methodology; visualization; writing—original draft; writing—review and editing. Mario Bardan Sarmiento : Investigation; methodology; writing—review and editing. Nicole Wernet : Methodology; writing—review and editing. Emily R. Troemel : Conceptualization; funding acquisition; resources; supervision; writing—original draft; writing—review and editing.
Conflict of Interest
The authors declare no conflict of interest.
Open Research
Data Availability Statement
The data that support the findings of this study are available from the corresponding author upon reasonable request.
Literature Cited
- Ashe, A., Bélicard, T., Le Pen, J., Sarkies, P., Frézal, L., Lehrbach, N. J., Félix, M. A., & Miska, E. A. (2013). A deletion polymorphism in the Caenorhabditis elegans RIG-I homolog disables viral RNA dicing and antiviral immunity. eLife , 2, e00994. https://doi.org/10.7554/eLife.00994
- Bakowski, M. A., Desjardins, C. A., Smelkinson, M. G., Dunbar, T. A., Lopez-Moyado, I. F., Rifkin, S. A., Cuomo, C. A., & Troemel, E. R. (2014). Ubiquitin-mediated response to microsporidia and virus infection in C. elegans. PLOS Pathogens , 10, e1004200. https://doi.org/10.1371/journal.ppat.1004200
- Batachari, L. E., Dai, A. Y., & Troemel, E. R. (2024). C. elegans RIG-I-like receptor DRH-1 signals via CARDs to activate anti-viral immunity in intestinal cells. bioRxiv: The Preprint Server for Biology , 2024.02.05.578694. https://www.biorxiv.org/content/10.1101/2024.02.05.578694v1
- Chen, Y. G., & Hur, S. (2022). Cellular origins of dsRNA, their recognition and consequences. Nature Reviews Molecular Cell Biology , 23, 286–301. https://doi.org/10.1038/s41580-021-00430-1
- Corsi, A. K., Wightman, B., & Chalfie, M. (2018). A Transparent window into biology: A primer on Caenorhabditis elegans. In WormBook: The Online Review of C. elegans Biology [Internet]. WormBook. https://www.ncbi.nlm.nih.gov/books/NBK299460/
- Current Protocols. (2006). Commonly Used Reagents. Current Protocols in Microbiology , 00, A.2A.1–A.2A.15. https://doi.org/10.1002/9780471729259.mca02as00
- Dimov, I., & Maduro, M. F. (2019). The C. elegans intestine: Organogenesis, digestion, and physiology. Cell and Tissue Research , 377, 383–396. https://doi.org/10.1007/s00441-019-03036-4
- Efstathiou, S., Ottens, F., Schütter, L. S., Ravanelli, S., Charmpilas, N., Gutschmidt, A., Le Pen, J., Gehring, N. H., Miska, E. A., Bouças, J., & Hoppe, T. (2022). ER-associated RNA silencing promotes ER quality control. Nature Cell Biology , 24, 1714–1725. https://doi.org/10.1038/s41556-022-01025-4
- Félix, M. A., Ashe, A., Piffaretti, J., Wu, G., Nuez, I., Bélicard, T., Jiang, Y., Zhao, G., Franz, C. J., Goldstein, L. D., Sanroman, M., Miska, E. A., & Wang, D. (2011). Natural and experimental infection of Caenorhabditis nematodes by novel viruses related to nodaviruses. PLoS biology , 9, e1000586. https://doi.org/10.1371/journal.pbio.1000586
- Félix, M. A., & Wang, D. (2019). Natural viruses of Caenorhabditis nematodes. Annual Review of Genetics , 53, 313–326. https://doi.org/10.1146/annurev-genet-112618-043756
- Franz, C. J., Renshaw, H., Frezal, L., Jiang, Y., Félix, M. A., & Wang, D. (2014). Orsay, Santeuil and Le Blanc viruses primarily infect intestinal cells in Caenorhabditis nematodes. Virology , 448, 255–264. https://doi.org/10.1016/j.virol.2013.09.024
- Gammon, D. B. (2017). Caenorhabditis elegans as an emerging model for virus-host interactions. Journal of Virology , 91, e00509–517. https://doi.org/10.1128/JVI.00509-17
- Guo, X., Zhang, R., Wang, J., Ding, S. W., & Lu, R. (2013). Homologous RIG-I–like helicase proteins direct RNAi-mediated antiviral immunity in C. elegans by distinct mechanisms. Proceedings of the National Academy of Sciences , 110, 16085–16090. https://doi.org/10.1073/pnas.1307453110
- Jiang, H., Chen, K., Sandoval, L. E., Leung, C., & Wang, D. (2017). An evolutionarily conserved pathway essential for Orsay virus infection of Caenorhabditis elegans. mBio , 8, e00940–e01017. https://doi.org/10.1128/mBio.00940-17
- Jiang, H., Franz, C. J., Wu, G., Renshaw, H., Zhao, G., Firth, A. E., & Wang, D. (2014). Orsay virus utilizes ribosomal frameshifting to express a novel protein that is incorporated into virions. Virology , 450, 213–221. https://doi.org/10.1016/j.virol.2013.12.016
- Jiang, H., Leung, C., Tahan, S., & Wang, D. (2019). Entry by multiple picornaviruses is dependent on a pathway that includes TNK2, WASL, and NCK1. eLife , 8, e50276. https://doi.org/10.7554/eLife.50276
- Jiang, H., Sandoval Del Prado, L. E., Leung, C., & Wang, D. (2020). Huntingtin-interacting protein family members have a conserved pro-viral function from Caenorhabditis elegans to humans. Proceedings of the National Academy of Sciences of the United States of America , 117, 22462–22472. https://doi.org/10.1073/pnas.2006914117
- Lažetić, V., Batachari, L. E., Russell, A. B., & Troemel, E. R. (2023). Similarities in the induction of the intracellular pathogen response in Caenorhabditis elegans and the type I interferon response in mammals. BioEssays: News and Reviews in Molecular, Cellular and Developmental Biology , 45, e2300097. https://doi.org/10.1002/bies.202300097
- Le Pen, J., Jiang, H., Di Domenico, T., Kneuss, E., Kosałka, J., Leung, C., Morgan, M., Much, C., Rudolph, K. L. M., Enright, A. J., O'Carroll, D., Wang, D., & Miska, E. A. (2018). Terminal uridylyltransferases target RNA viruses as part of the innate immune system. Nature Structural & Molecular Biology, 25, 778–786. https://doi.org/10.1038/s41594-018-0106-9
- Liu, W. H., Lin, Y. L., Wang, J. P., Liou, W., Hou, R. F., Wu, Y. C., & Liao, C. L. (2006). Restriction of vaccinia virus replication by a ced-3 and ced-4-dependent pathway in Caenorhabditis elegans. Proceedings of the National Academy of Sciences of the United States of America , 103, 4174–4179. https://doi.org/10.1073/pnas.0506442103
- Lu, R., Maduro, M., Li, F., Li, H., Broitman-Maduro, G., Li, W., & Ding, S. (2005). Animal virus replication and RNAi-mediated antiviral silencing in C. elegans. Nature , 436, 1040–1043. https://doi.org/10.1038/nature03870
- Lu, R., Yigit, E., Li, W. X., & Ding, S. W. (2009). An RIG-I-Like RNA helicase mediates antiviral RNAi downstream of viral siRNA biogenesis in Caenorhabditis elegans. PLOS Pathogens , 5, e1000286. https://doi.org/10.1371/journal.ppat.1000286
- Nyaanga, J., Crombie, T. A., Widmayer, S. J., & Andersen, E. C. (2021). easyXpress: An R package to analyze and visualize high-throughput C. elegans microscopy data generated using CellProfiler. PLOS ONE , 16, e0252000. https://doi.org/10.1371/journal.pone.0252000
- Pukkila-Worley, R., & Ausubel, F. M. (2012). Immune defense mechanisms in the Caenorhabditis elegans intestinal epithelium. Current Opinion in Immunology , 24, 3–9. https://doi.org/10.1016/j.coi.2011.10.004
- Reddy, K. C., Dror, T., Sowa, J. N., Panek, J., Chen, K., Lim, E. S., Wang, D., & Troemel, E. R. (2017). An intracellular pathogen response pathway promotes proteostasis in C. elegans. Current Biology: CB , 27, 3544–3553.e5. https://doi.org/10.1016/j.cub.2017.10.009
- Reddy, K. C., Dror, T., Underwood, R. S., Osman, G. A., Elder, C. R., Desjardins, C. A., Cuomo, C. A., Barkoulas, M., & Troemel, E. R. (2019). Antagonistic paralogs control a switch between growth and pathogen resistance in C. elegans. PLOS Pathogens , 15, e1007528. https://doi.org/10.1371/journal.ppat.1007528
- Rivera, D. E., Lažetić, V., Troemel, E. R., & Luallen, R. J. (2022). RNA Fluorescence in situ Hybridization (FISH) to visualize microbial colonization and infection in Caenorhabditis elegans intestines. Journal of Visualized Experiments: JoVE , 185, https://doi.org/10.3791/63980
- Sandoval, L. E., Jiang, H., & Wang, D. (2019). The dietary restriction-like Gene drl-1, which encodes a putative serine/threonine kinase, is essential for Orsay virus infection in Caenorhabditis elegans. Journal of Virology , 93, e01400–e01418. https://doi.org/10.1128/JVI.01400-18
- Schott, D. H., Cureton, D. K., Whelan, S. P., & Hunter, C. P. (2005). An antiviral role for the RNA interference machinery in Caenorhabditis elegans. Proceedings of the National Academy of Sciences of the United States of America , 102, 18420–18424. https://doi.org/10.1073/pnas.0507123102
- Son, K. N., Liang, Z., & Lipton, H. L. (2015). Double-stranded RNA is detected by immunofluorescence analysis in RNA and DNA virus infections, including those by negative-stranded RNA viruses. Journal of Virology , 89, 9383–9392. https://doi.org/10.1128/JVI.01299-15
- Sowa, J. N., Jiang, H., Somasundaram, L., Tecle, E., Xu, G., Wang, D., & Troemel, E. R. (2020). The Caenorhabditis elegans RIG-I homolog DRH-1 mediates the intracellular pathogen response upon viral infection. Journal of Virology , 94, e01173–e01219. https://doi.org/10.1128/JVI.01173-19
- Stiernagle, T. (2006). Maintenance of C. elegans. In WormBook: The Online Review of C. elegans Biology [Internet]. WormBook. https://www.ncbi.nlm.nih.gov/books/NBK19649/
- van den Hoogen, J., Geisen, S., Routh, D., Ferris, H., Traunspurger, W., Wardle, D. A., de Goede, R. G. M., Adams, B. J., Ahmad, W., Andriuzzi, W. S., Bardgett, R. D., Bonkowski, M., Campos-Herrera, R., Cares, J. E., Caruso, T., de Brito Caixeta, L., Chen, X., Costa, S. R., Creamer, R., … Crowther, T. W. (2019). Soil nematode abundance and functional group composition at a global scale. Nature , 572, 194–198. https://doi.org/10.1038/s41586-019-1418-6
- Wilkins, C., Dishongh, R., Moore, S. C., Whitt, M. A., Chow, M., & Machaca, K. (2005). RNA interference is an antiviral defence mechanism in Caenorhabditis elegans. Nature , 436, 1044–1047. https://doi.org/10.1038/nature03957
- Zhou, Y., Chen, H., Zhong, W., & Tao, Y. J. (2024). Collagen and actin network mediate antiviral immunity against Orsay virus in C. elegans intestinal cells. PLOS Pathogens , 20, e1011366. https://doi.org/10.1371/journal.ppat.1011366
Internet Resources
Fiji software download source.