Mouse and Rat Anesthesia and Analgesia
Sang Su Oh, Sang Su Oh, Heather L. Narver, Heather L. Narver
Abstract
Anesthesia and analgesia play pivotal roles in ethically and humanely using animal models in research, especially concerning mice and rats. These rodent species, extensively utilized in scientific investigations due to their genetic resemblance to humans, serve as invaluable tools for studying diseases and testing treatments. Proper anesthesia and analgesia not only prioritize animal welfare but also heighten experimental validity by minimizing stress-induced physiological responses. Recent years have seen remarkable advancements in anesthesia for mice and rats. The focus has shifted away from the ‘one size fits all’ toward tailoring anesthesia protocols, considering factors like age, strain, and the nature of the experimental procedure. The use of inhalation agents such as isoflurane and sevoflurane is often preferred due to their rapid induction and recovery characteristics, allowing precise control over anesthesia depth. However, refinements in injectable anesthetic agents also provide researchers the flexibility to select suitable agents based on study requirements. Additionally, progress in analgesic techniques has led to effective pain management strategies for these rodents. Common analgesics such as nonsteroidal anti-inflammatory drugs (NSAIDs), opioids, and local anesthetics are administered to alleviate pain and discomfort. However, standard practice also involves continuous monitoring of animals' behavior and physiological parameters, ensuring timely adjustments in analgesic regimens for optimal pain relief without compromising experimental outcomes. By integrating tailored anesthesia and analgesia protocols into the experimental design, researchers uphold high animal welfare standards while obtaining reliable scientific data. This contributes significantly to advancing medical knowledge and therapeutic interventions with reproducible results. Published 2024. This article is a U.S. Government work and is in the public domain in the USA. Current Protocols published by Wiley Periodicals LLC.
Basic Protocol 1 : Injectable anesthesia for mouse and rat
Basic Protocol 2 : Inhalant anesthesia using isoflurane for mouse and rat
Basic Protocol 3 : Analgesia for mice and rats
INTRODUCTION
Managing anesthesia and analgesia in rodents is challenging due to their small size and varying sensitivities to anesthetics and analgesics. Often, one focuses on the surgical procedure rather than pre- and post-operative procedures, i.e., selecting the appropriate anesthetic and monitoring the animal's physiological status during and after the surgery. In this context, it is vital to emphasize proper preparation, particularly if one is performing multiple surgeries. Investigators should become familiar with the various types of anesthetic agents, understand their physiological effects, and be aware of the associated risks (margin of safety) of the agent chosen. This, together with sterile surgical techniques and post-operative care, plays a pivotal role in ensuring the successful creation of the surgical model (Davis, 2008).
This article provides three protocols for use in mice and rats. Basic Protocol 1 discusses injectable anesthesia. Basic Protocol 2 concentrates on inhalant anesthesia, and Basic Protocol 3 focuses on analgesia. The accompanying commentary offers insights into the advantages and disadvantages of each anesthetic method, along with practical tips for effective animal monitoring. Additionally, the commentary discusses key aspects of rodent physiology (Table 1) that must be considered to avoid common problems associated with anesthesia in rodents. Furthermore, the benefits of using analgesia in rodents are presented, but strategies are provided for situations in which commonly used analgesic agents may not be suitable (Davis, 2008).
Mouse | Rat | |
---|---|---|
Temperature (°C) | 37-37.2 | 37.5 |
Heart rate (beats/min) | 320-840 | 300-500 |
Respiratory rate (beats/min) | 163 | 85 |
Tidal volume (ml)b | 0.2 | 1.5 |
Minute ventilation (ml)b | 24 | 200 |
Body surface area (m2) | 0.007 | 0.025 |
Blood volume (ml/100 mg) | 5.58 | 6 |
- a Published parameters vary, which probably reflects differences in strain, age, and gender of the species (Andersen et al. 2015, Nair & Jacob 2016).
- b Tidal volume is the volume of gas drawn into the respiratory tract with each breath. Minute ventilation is the volume of gas breathed in 1 min (tidal volume × respiratory rate). Rodents maintain minute ventilation by high respiratory rate and low tidal volume. During anesthesia, both parameters fall, causing a decrease in minute ventilation.
NOTE : All protocols using live animals must first be reviewed and approved by the appropriate Animal Care and Use Committee and must follow regulations for the care and use of laboratory animals.
NOTE for Basic Protocols 1 and 2: An external heat source should be provided to all anesthetized animals to maintain body temperature. This can be accomplished by using feedback-controlled warning pads, Infrared warming pads, recirculating warm water blankets, forced warm air units, or pocket warmers. If pocket warmers are used, Do NOT place hand warmer(s) in the cage without first wrapping them. Also, electric heating pads and heat lamps are not recommended because of their potential to cause burn injuries.
Basic Protocol 1: INJECTABLE ANESTHESIA FOR MOUSE AND RAT
Mice and rats have high metabolic rates. As such, they require higher anesthetic dosages than larger animals with low metabolic rates to achieve an effective level of anesthesia. In addition, the duration of anesthesia is typically shorter (20-30 min), and mice and rats are less likely to survive respiratory arrest from overdosage. The high metabolic rate also increases the risk of hypothermia and dehydration due to exposed membranes and small body size (Davis, 2008).
Injectable anesthetics are useful when multiple mice or rats are anesthetized simultaneously or serially to allow short surgical procedures to be performed on a group of animals. Injectable anesthetics can be rapidly administered by laboratory personnel after a relatively short training period. Some of these drugs are convenient to use since they are readily available as noncontrolled substances and require no special equipment. Regardless of which injectable anesthetic is used, each animal must be weighed and dosed accordingly. Rodents do not require fasting prior to administration of anesthetic as they cannot vomit. However, once the animal loses consciousness, an external heat source should be used to help maintain body temperature (Davis, 2008).
CAUTION : Once a chemical anesthetic is given, it is difficult to adjust the dose. In some animals, the standard dose may be inefficient in achieving a stable plane of anesthesia, while in other animals, the same dose may be excessive and may lead to mortality. If a repeated dose is needed, it should be around 10%-25% of the initial dose, but be aware that even though a lower dose is used, it can increase the risk of adverse side effects, including delayed toxicity or overdose and death.
Materials
- Laboratory mice or rats
- Anesthetic of choice (Table 2)
- Petroleum-based artificial tear ointment
- Lactated Ringer's solution or 0.9% Normal Saline, warmed to 36-38°C
- For the surgeon (PPE):
- Clean laboratory coat
- Face mask
- Headcover
- Sterile gloves
Agent | Species | Dosage (mg/kg)a | Route | Duration of anesthesia (min)b |
---|---|---|---|---|
Pentobarbitalc | Mouse | 40-70 | IP | 10-300 |
Rat | 40-50 | IP | 80-95 | |
Ketamine/xylazined | Mouse | 60-100 ket + 5-10 xyl | IP | 30-45 |
Rat | 40-90 ket + 5-10 xyl | IP | 45-90 | |
Ketamine/xylazine/acepromazinee | Mouse | 80-100 ket + 5-10 xyl + 1 ace | IP | 40 |
Rat | 31.25 ket + 6.25 xyl + 1.25 ace | IP | Unproven | |
Ketamine/dexmedetomidinef | Mouse | 50-75 ket + 0.5-1 dex | IP | 20-30 |
Rat | 75 ket + 0.5 dex | IP | 120 | |
Atipamezole | 0.1-1 | IP, SC | Reversal agent for alpha-2 agonists | |
Tribromoethanolg (Avertin or TBE) |
Mouse | 125-250 | IP | 30-45 |
Rat | 300 | IP | 12-20 | |
Atropineh | Mouse | 0.04-0.1 | SC | Anticholinergic |
Rat | 0.05 | SC | Anticholinergic | |
Bupivacainei | Mouse/rat | 0.25% solution. Local infiltration or drop-wise administration to incision site | NA | |
Lidocainei | Mouse/rat | 1% solution. Local infiltration or drop-wise administration to incision site (or cream- EMLA®) | NA |
- a
Dosages are from a variety of sources. The dose may need to be adjusted for individual situations. Abbreviations: IP, intraperitoneally; SC, subcutaneously.
- b
Duration of surgical anesthesia (unconscious, nonresponsive to painful stimuli) is not the same as “sleep time” (quiet, not moving, but responsive to stimuli), which is generally much longer.
- c
This is a controlled substance. Barbiturate classification (long, short, ultrashort) is misleading, as species differences in barbiturate pharmacokinetics are responsible for significant variations in the duration of action and may have a narrow margin of safety in most animal species. Given their lack of analgesic properties, they are often combined with sedatives or tranquilizers to provide deep anesthesia and smooth recovery.
- d
Ketamine and xylazine can be safely mixed and given as a single injection for long-term procedures. After mixing, dilute 1:10 (v/v) with sterile saline. However, in certain rodent studies, this mixture may not produce surgical-plane anesthesia for major procedures, but this mixture in rats is more reliable than in mice. If redosing, use 1/3 dose of ketamine alone—may lose surgical anesthesia. Xylazine may be reversed with Atipamezole. Ketamine is a controlled drug. Caution: Some strains of rats are at increased risk for developing post-anesthetic corneal lesions with this drug combination (Turner & Albassam, 2005).
- e
Acepromazine should be stored in a dark place protected from light, ensuring that the mixture is also shielded from exposure to light. May not produce surgical-plane anesthesia for major procedures. If redosing, use 1/3 dose of ketamine alone. Xylazine may be reversed with Atipamezole. Do not use in preweanling animals. Ketamine is a controlled drug.
- f
to surgical anesthesia. If redosing, use 1/3 dose of ketamine alone—may lose surgical anesthesia. Dexmedetomidine may be reversed with Atipamezole. Ketamine is a controlled drug.
- g
Tribromoethanol (TBE) is an injectable anesthetic agent with minimal cardiodepressive effect, once manufactured specifically for anesthetic use under the trade name Avertin. But the manufactured product is no longer available. As a result, investigators must make their own solutions from non-pharmaceutical-grade chemicals (PGCs). The use of non-PGCs must be described and justified in animal study proposals and approved by IACUC. Make a 100% (w/v) stock solution by dissolving 5 g of 2,2,2-tribromoethanol in 5 ml 2-methyl-2-butanol (tert-amyl alcohol). The stock solution is light-sensitive and evaporates rapidly. Do not leave the bottle open longer than is necessary. Label, date, and refrigerate (4°C) in a tightly sealed, dark amber or foil-covered bottle. Unused stock solution refrigerated at 4°C should be discarded after 6 months. Alternatively, the stock solution can be made and aliquoted into 1 ml snap cap tubes. The tubes containing the stock solution are then stored, wrapped in foil (to protect it from the light), and placed under an inert gas (nitrogen or argon) at –70°C. Stock solution stored frozen at –70°C under inert gas is stable indefinitely. A working solution (anesthetic solution) of TBE is prepared by diluting the stock solution to isotonic saline (0.9% NaCl) and sterilized by filtration (0.2-micron filter) into a sterile injection vial. Working solutions are kept in the dark at 4°C and utilized within thirty (30) days of preparation. Working solutions must be labeled with the expiration date of the solution. CAUTION: Stored solutions often deteriorate to irritant solutions that cause peritonitis, ileus, and/or death following IP administration. Repeated injections of Tribromoethanol (TBE) escalate the risk of significant peritonitis, ileus, and potential mortality. Indicative of toxic degradation, a yellowing solution necessitates immediate replacement of the stock. Additionally, any solution exhibiting crystal formation or precipitate must be promptly discarded. While TBE has typically been used for non-survival surgeries, for survival surgeries, it is crucial to perform daily monitoring over several days to promptly identify any possible complications arising from TBE.
- h
Atropine is an anticholinergic, not an anesthetic. Atropine blocks acetylcholine at muscarinic receptors. Desirable effects include a reduction in bronchial secretions and protection of the heart from vagal stimulation, which may occur during surgical procedures. If used, it should be given 5 to 10 min before the anesthetic agent.
- i
Bupivacaine/lidocaine applied to the incision site during or after closure of the incision augments analgesia by providing local anesthesia at the site of the incision. Bupivacaine has a slower onset within 1-15 min but a longer duration of action, lasting from 4 to 12 hr. In contrast, Lidocaine has a rapid onset within 1-5 min but a shorter duration, typically lasting 1.5-2 hr. Coating the tips of the ear bars with lidocaine gel or administration of analgesic at least 5-10 min prior to placing mice onto stereotaxic device will provide analgesia during this period.
NOTE : Start with sterile surgical instruments and employ a “tips-only” technique. This technique sterilizes only the tips of the instruments between each procedure using a hot bead sterilizer. In surgery, the “tips-only” approach ensures that only the sterile working ends of the instruments are used to manipulate the surgical field. This prevents any contact between the non-sterile parts of instruments and gloves with the surgical field. The method is particularly useful when performing surgery alone and dealing with multiple animals and non-sterile equipment. After using a hot bead sterilizer between procedures, it is crucial to cool the instruments before they come into contact with tissues.
1.Prepare the operating area so it is clean and uncluttered and provides adequate room for instruments, equipment, a warming device, and, if required, a stereotaxic apparatus. Ensure that the area is well-lit.
2.Set up a warming device and turn it on. If required, cover it with a clean pad or drape.
3.Determine the animal's weight by placing it in a tared container on a scale.
4.Return the animal to its cage.
5.Calculate the appropriate dosage of the chosen anesthetic using Table 2.Fill either a 1- or 3-ml syringe with selected anesthetics.
6.Manually restrain the animal and inject the selected dose intraperitoneally (Donovan & Brown, 2005).
7.Return the animal to an empty cage and monitor for the depth of anesthesia (e.g., pedal withdrawal reflex, loss of righting reflex, loss of palpebral blink reflex, rate and depth of respiration). Do not put the animal in a cage containing other animals.
8.Apply a petroleum-based artificial tear ointment to the eyes to prevent corneal desiccation since anesthesia will abolish the blink reflex. The application of eye ointment should be performed before head-shaving in the case of brain surgery.
9.Optional : To maintain hydration, postoperatively, inject warmed (35-36°C) lactated Ringer's solution or 0.9% NaCl (saline) under the skin (subcutaneously) between the shoulder blades at 10-40 mg/kg for mouse (do not exceed 1 ml per site) and 5-10 ml/kg for rat (do not exceed 5 ml per site).
10.Maintain continuous monitoring throughout anesthesia and recovery, ensuring regular assessment and documentation of anesthetic depth and respiratory rate every 15 min. Documentation facilitates recognition of trends or patterns to inform and refine subsequent anesthetic use.
Basic Protocol 2: INHALANT ANESTHESIA USING ISOFLURANE FOR MOUSE AND RAT
The most consistent anesthetic protocols for rodents rely on inhalant anesthesia, with isoflurane being the most frequently employed inhalant agent. There are two primary advantages to utilizing inhalant anesthetics: 1) activating inhibitory synapses and 2) rapid uptake. It is well-established that inhalant agents activate both gamma-aminobutyric acid (GABA) and glycine receptors while inhibiting the NMDA receptor. This combined action results in the inhibition of central nervous system neural activity, leading to the characteristic signs of immobility, hypnosis, and amnesia associated with anesthesia. Thanks to their impact on multiple receptors, inhalant anesthetics exhibit an exceptionally steep dose-response curve, allowing nearly 100% of animals to attain the desired surgical plane. Furthermore, inhalant agents have a low blood solubility, which facilitates rapid drug uptake from the alveoli and efficient distribution across the blood-brain barrier. This results in swift anesthetic induction, as well as rapid removal of the inhalant agents from the central nervous system at the conclusion of a procedure, and the ability to make subtle adjustments to anesthetic depth during the procedure. In contrast, similar rapid changes in central nervous system anesthetic levels are generally not feasible with routine injectable anesthetic administration, as it necessitates liver metabolism before excretion.
Nonetheless, it is important to acknowledge certain disadvantages associated with inhalant anesthetics in rodent models. Rapid changes in anesthetic depth necessitate vigilant animal monitoring due to the potential for swift fluctuations, and surgical teams must possess the proficiency to effectively administer and maintain the delivery of inhalant anesthetic agents. Additionally, the use of a precision vaporizer, regularly calibrated to maintain accuracy, is indispensable for the precise administration of inhalant anesthetics, albeit incurring an extra cost compared to injectable anesthetics. It is worth noting that the release of waste anesthetic gas into the environment poses an occupational health risk for individuals involved in anesthesia procedures. Therefore, the implementation of a scavenger system (either active or passive) and appropriate air ventilation within procedure rooms becomes imperative for safety.
The administration of volatile anesthetic agents to rodents is typically accomplished using a face mask/or nosecone, primarily because the animals’ small size makes endotracheal intubation more challenging in comparison to larger species such as dogs or cats. The use of a face mask/or nosecone is relatively straightforward, and one can quickly become proficient in its application. In certain cases, custom-designed facemasks may be required, especially when they are intended for use alongside other devices, such as bite bars for stereotaxic surgery. Additionally, commercial face masks designed for this purpose are available for purchase (see Materials below).
Many combined injectable anesthetic agents, such as ketamine/alpha-2 adrenergic agonists and local anesthetics, are recognized for their analgesic properties. In contrast, inhalant anesthetics lack these analgesic effects, necessitating careful consideration. Hence, as animals transition from anesthesia to the ability to perceive pain, it is imperative to have an analgesia protocol in place to ensure a seamless recovery process before discontinuing the use of inhalant anesthetics. Administering pre-operative analgesics is highly recommended (see Basic Protocol 3), serving the dual purpose of averting pain during the recovery phase and reducing the need for inhalant anesthesia, thereby mitigating subsequent risks of respiratory suppression and hypotension. Additionally, pre-operative analgesics contribute to diminishing the stress associated with tank induction procedures for the animals.
CAUTION : Use of a face mask instead of endotracheal intubation makes positive pressure ventilation less effective in the case of an emergency. An endotracheal tube (ETT) allows control of the animal's airway in the event of cardiac or respiratory arrest, as the animal can be artificially ventilated. However, a high level of technical competence is required to consistently insert an ETT, and attempts to intubate rodents, even by experienced personnel, often result in trauma to the oropharynx, larynx, trachea, and occasionally the esophagus.
Materials
- Isoflurane bottle and bottle adaptor for refill; 250-ml bottles are often used.
- Precision isoflurane vaporizer (Fig. 1)
- Laboratory mice or rats
- Petroleum-based artificial tear ointment
- Lactated Ringer's solution or 0.9% Normal Saline, warmed to 36-38°C (optional)
- For the surgeon (PPE):
- Clean laboratory coat
- Face mask
- Headcover
- Sterile gloves
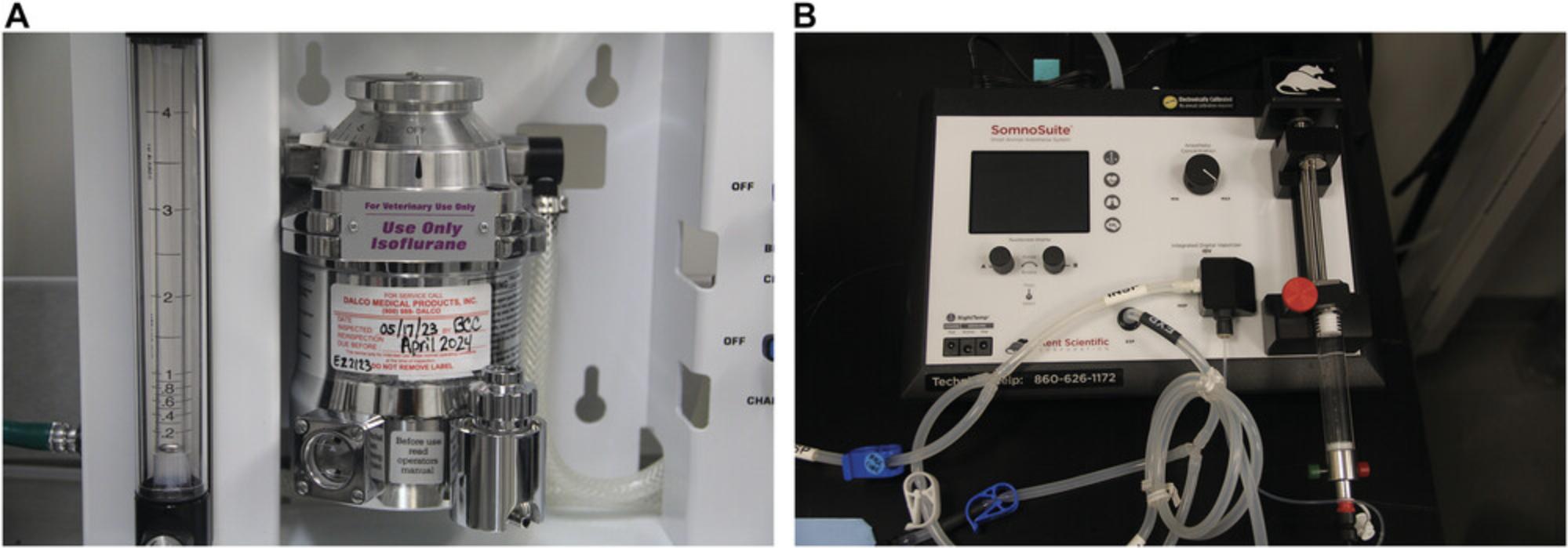
- Warming device and optional monitoring probe 36-38°C
- Optional monitoring devices: Heart rate, respiratory rate, blood pressure, oxygenation (%SpO2), and electrocardiogram (ECG)
- Sterile surgical instruments
- Sterile drape (optional if performing tips only aseptic techniques)
- Oxygen tank with flowmeter or central supply system (Fig. 2A and B)
- Induction chamber with inlet and outlet ports (1-2 L size for mouse, or 3-5 L for rat)
- Scavenger system: active (Fig. 3) or passive circuit with activated charcoal canister (E-Z Systems Inc. or VetEquip Inc.)
- Commercial rodent face mask (optional; Kent Scientific or SurgiVet) or stereotaxic specific face mask (Fig. 4)
- Cages for mice or rats
- Laboratory scale or balance (capacity 800 g; accuracy 0.1 g)
- or 3-ml syringe
- 25-27 G needle for mice
- 23-25 G needle for rats
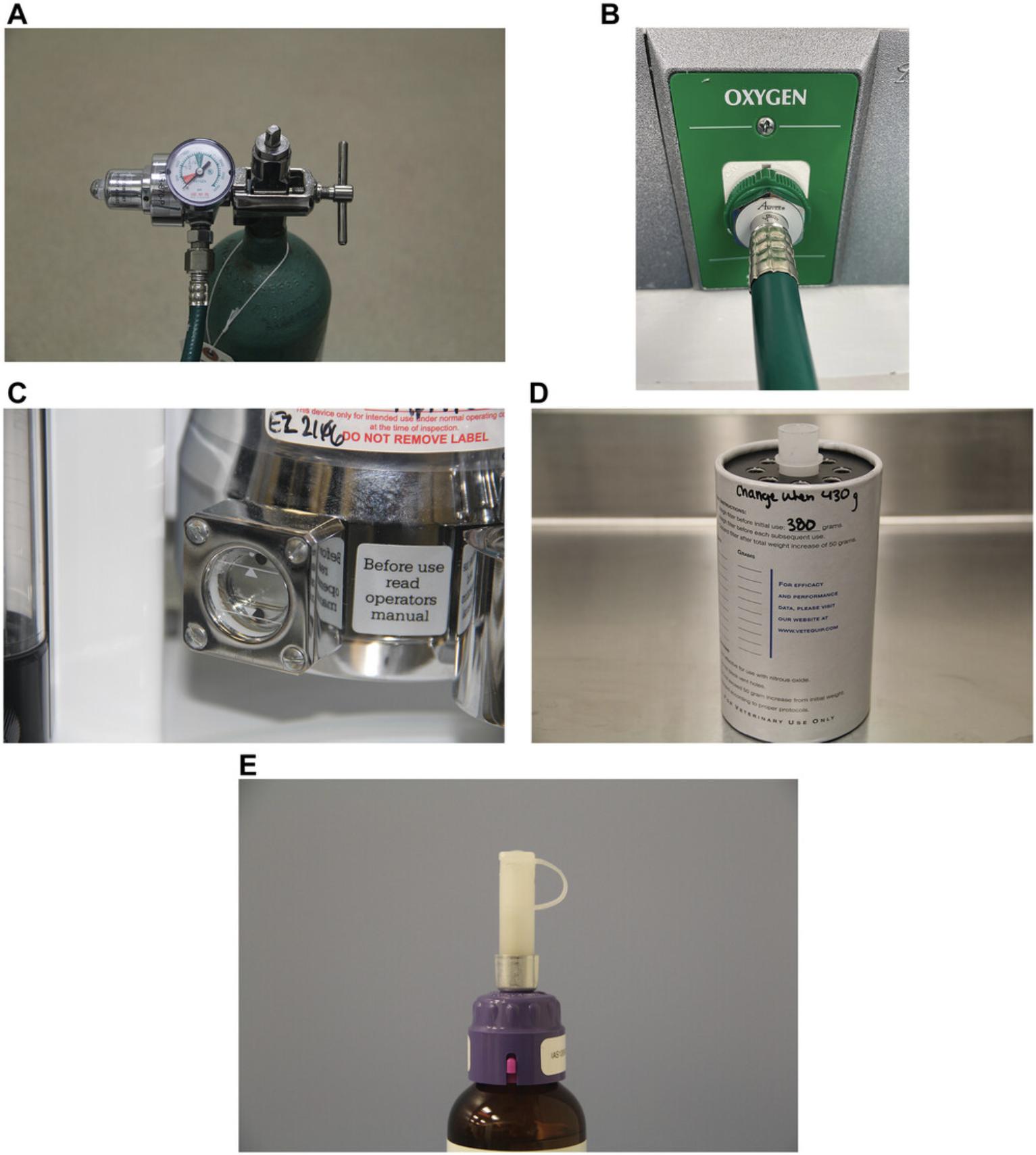
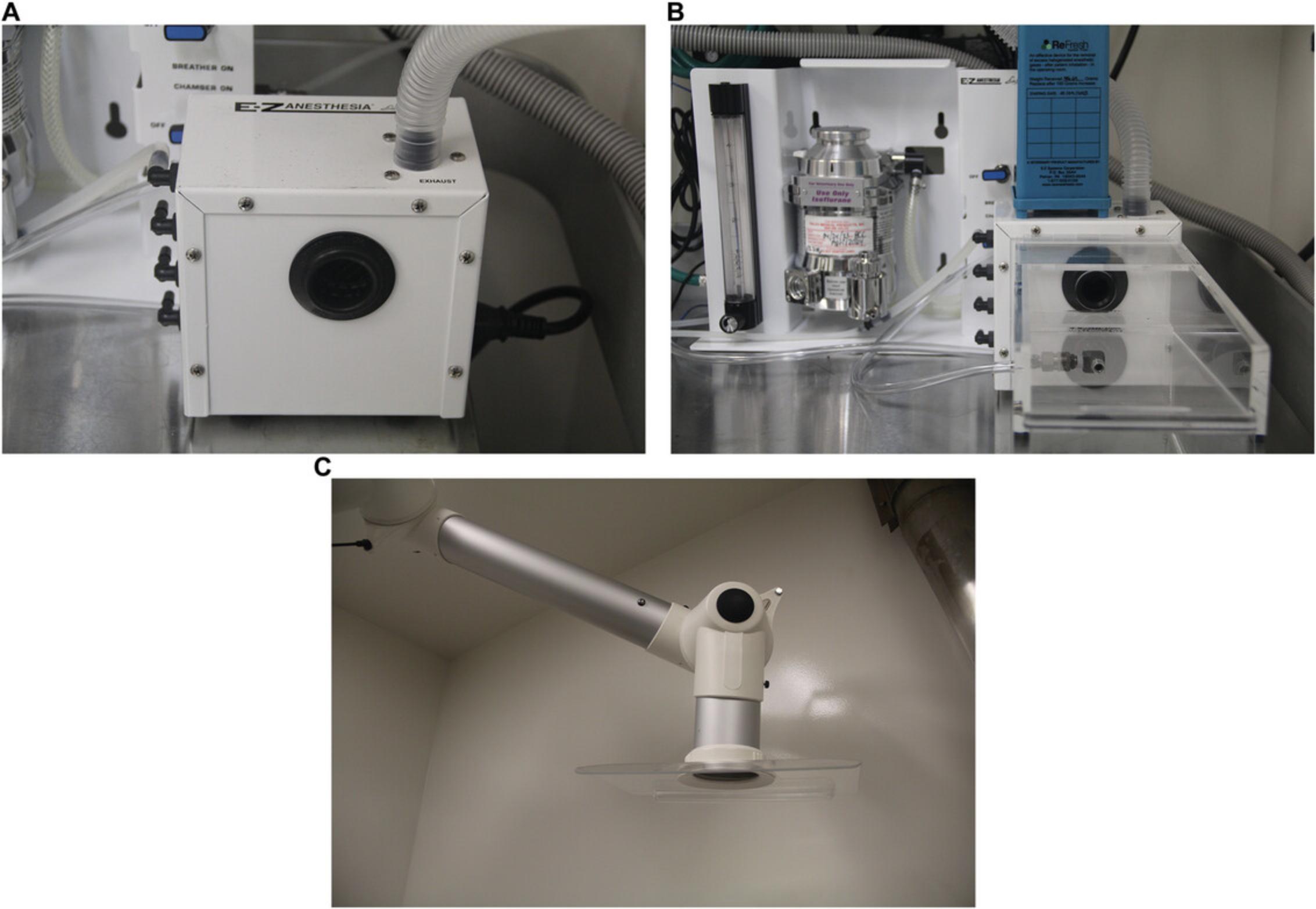
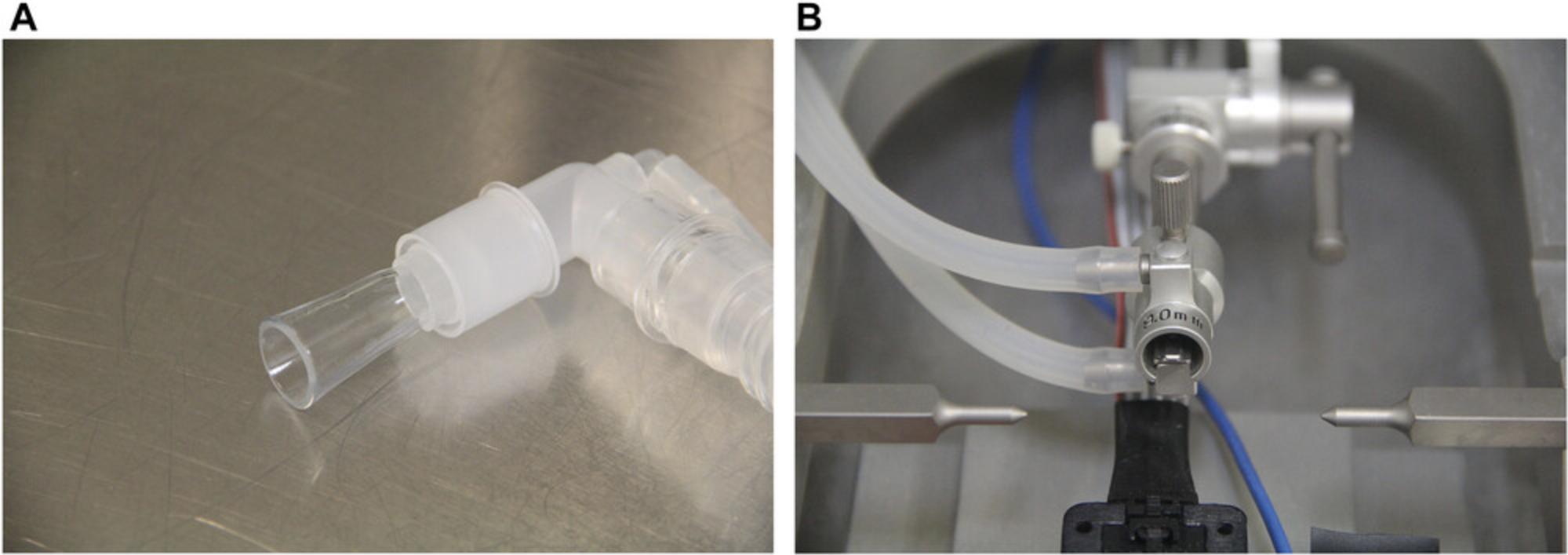
NOTE : Start with sterile surgical instruments and employ a “tips-only” technique. This technique sterilizes only the tips of the instruments between each procedure using a hot bead sterilizer. In surgery, the “tips-only” approach ensures that only the sterile working ends of the instruments are used to manipulate the surgical field. This prevents any contact between the non-sterile parts of instruments and gloves with the surgical field. The method is particularly useful when performing surgery alone and dealing with multiple animals and non-sterile equipment. After using a hot bead sterilizer between procedures, it is crucial to cool the instruments before they come into contact with tissues.
CAUTION : All procedures with inhalant agents require a scavenging device for expired anesthetic gases. Frequently, a chemical fume hood, down draft table, or safety cabinet is helpful for the continuous exhaust of anesthetic gases away from personnel (see Critical Parameters). Prior to first use, the activated charcoal canister should be weighed on a gram scale, and the initial weight should be recorded on the canister. Ideally, the canister should be weighed after each surgical procedure and replaced after the manufacturer's suggested weight is achieved. The majority of canisters require replacement when they reach a weight of 50 g more than the beginning weight. Also, depending on the location of the gas exhaust port, canisters should always be suspended upright, allowing excess gases to flow through the bottom/top of the canister. Do not lay the charcoal canisters on their side during use. Additionally, commercially available anesthetic equipment is equipped with an O2 flush valve to clear the induction chamber, minimizing pollution upon opening. It is crucial to note that the O2 flush valve should never be employed when an animal is connected to a non-rebreathing circuit (Dyson et al., 2023).
Prepare work area
Prepare the operating area so it is clean, uncluttered, and provides adequate room for instruments, equipment, heat support, and, if required, a stereotaxic apparatus (Fig. 5). Ensure that the area is well-lit.
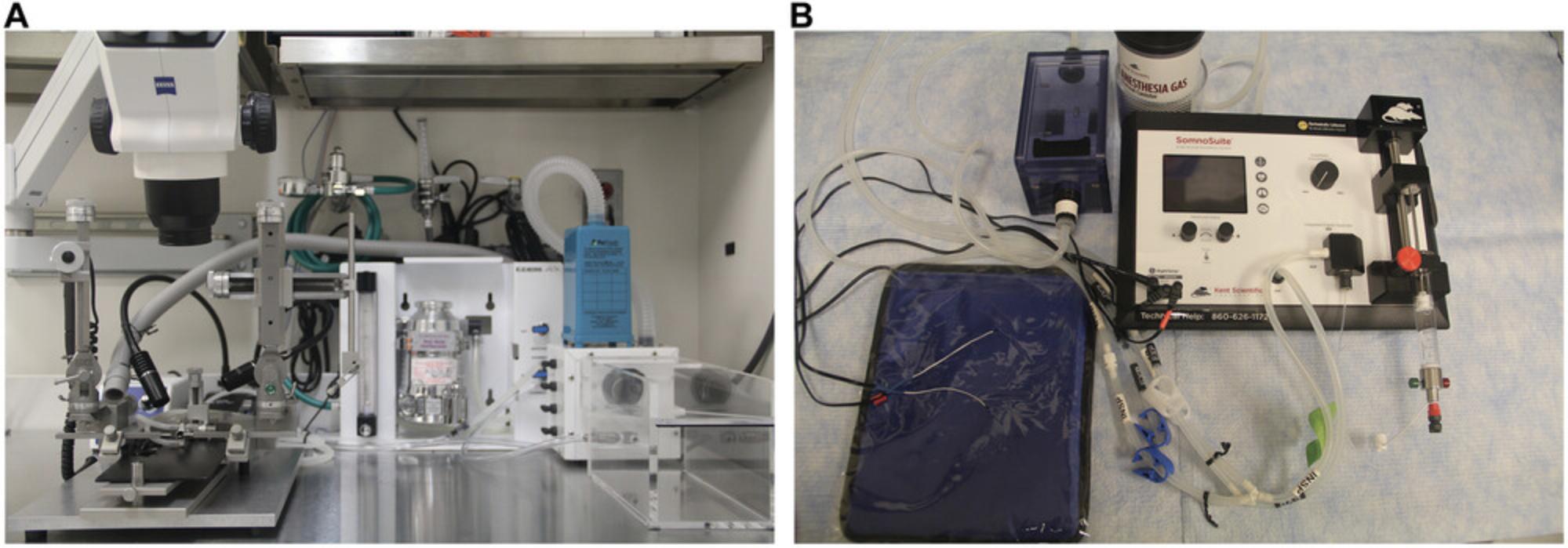
Prepare induction
1.Plug in and turn on a warming device. If using a recirculating warm water blanket, cover it with a drape. The warming device will ultimately go under the anesthetized rodent.
2.Check and connect oxygen tank to vaporizer.
3.Check scavenger system (Fig. 2D).
4.Check isoflurane level and pour isoflurane into the vaporizer to the fill line as necessary (Fig. 2C).
5.Connect the vaporizer to an induction chamber.
6.The all-anesthesia circuits should be tested and inspected to ensure that O2/isoflurane supplies are adequate, properly connected, and functioning.
7.Turn the oxygen valve on to provide high flow (100% oxygen) to pre-charge the chamber.
Deliver anesthesia
8.Retrieve the animal from its cage and place it in the induction chamber. Expose the animal to 100% oxygen for 2-3 min, and then adjust the precision vaporizer (dial) to provide 4%-5% isoflurane.
9.Monitor the animal until it becomes ataxic, recumbent, with a steady, slow respiratory rate, and immobile, which signifies the onset of anesthesia.
10.Remove the animal from the induction chamber and place the face mask or nosecone over the muzzle of the animal to maintain anesthetic delivery.
11.Connect the tubing from the induction chamber to the face mask or nosecone, adjust the dial on the vaporizer to deliver 1%-3% isoflurane, and adjust the flowmeter to deliver 1 L of oxygen per minute for maintenance anesthesia.
12.Apply a petroleum-based artificial tear ointment to the eyes to prevent corneal desiccation.
13.Assess the depth of anesthesia, including factors such as respiration rate, depth, and response to toe pinch (pedal withdrawal reflex), before making the surgical incision. Adjust the vaporizer dial to 1%-3% to maintain the appropriate anesthetic level for the duration of the surgery.
14.Optional : To maintain hydration, postoperatively, inject warmed (35-36°C) lactated Ringer's solution or 0.9% NaCl (saline) under the skin (subcutaneously) between the shoulder blades. Use 10-40 mg/kg for mouse (do not exceed 1 ml per site) and 5-10 ml/kg for rat (do not exceed 5 ml per site).
15.Maintain continuous monitoring throughout anesthesia and recovery, ensuring regular assessment and documentation of anesthetic depth and respiratory rate at intervals of no less than every 15 min. Documentation facilitates recognition of trends or patterns to inform and refine subsequent anesthetic use.
16.At the end of the procedure, turn the vaporizer off and deliver 100% oxygen to the animal for ∼5 min.
Basic Protocol 3: ANALGESIA FOR MICE AND RATS
General anesthesia, while inducing a state of unconsciousness, selectively depresses the cerebral cortex, leaving the rest of the nervous system largely unaffected. In surgical contexts, the initiation of a scalpel incision stimulates primary sensory afferents, transmitting nociceptive impulses to the spinal cord. This process sensitizes the nociceptor system to further input from injured tissue, persisting beyond the original stimulus. Moreover, this pain activates the sympathetic system, leading to an increase in epinephrine, which heightens the responsiveness of injured neurons to stimulation. Furthermore, a reflexive increase in muscle tension around the site of injury adds to the pain. Pain associated with surgery can also lead to disturbances in the rodents’ overall physiological functions, including the immune system, reduced food and water intake, alterations in normal behavior, and even changes in sleep patterns, which can result in persistent pain due to central nervous system sensitization.
Analgesia, in its strictest sense, signifies the absence of pain. In practical terms, it involves an effort to reduce the intensity of perceived pain. The objective is to alleviate pain as much as possible without excessively depressing the animal. However, once the effects of anesthesia wear off and consciousness returns, animals may still experience pain unless it has been appropriately managed. It is worth noting that even when an animal is under anesthesia, nociceptors can be activated, leading to post-procedural hyperalgesia or allodynia. The duration and intensity of post-procedural pain vary based on the type of procedure and pain model used, with the most intense pain typically occurring between 4 and 24 hr after surgery (Davis, 2008).
Preventing or preemptively managing nociception is a critical strategy to minimize post-operative pain. Preventive analgesia, administered before a procedure, helps reduce the need for inhalant anesthetics. However, relying solely on preventive analgesia or a single analgesic post-surgery may not suffice. Multimodal analgesia, involving different analgesic agents, targets various sources of pain. This approach allows for lower analgesic doses, minimizing side effects and enhancing pain relief. Timing is vital; analgesic coverage during anesthetic emergence and the postoperative period is essential for effective pain management.
The selection of an appropriate analgesic regimen depends on several factors, including the type of procedure performed, the nature of the pain (in terms of severity and duration), and how it might affect the research objectives. Various options, such as topical, regional, central, and peripherally acting analgesics, are available. It is essential to consider the species and strain of the animal, the choice of anesthetic, and the invasiveness of the procedure when making analgesic choices. There is no one-size-fits-all plan for treating pain in all surgical or invasive procedures involving these animals, underscoring the need for collaboration between researchers and veterinarians to create customized analgesic plans for each study. For instance, if isoflurane is used, analgesics should be administered before surgery, while injectable anesthetics can be given at the end of the procedure. In minor surgeries like jugular catheterization or subcutaneous implantation, a one-time administration of an analgesic with a topical anesthetic, such as lidocaine, on the incision line may be sufficient. However, in the case of major surgeries, administration of analgesics for 48-72 hr or a combination of different analgesics, such as NSAIDs and opioids, may be necessary. Ensuring proper post-operative care is not only essential for the welfare of these animals but also a fundamental aspect of sound scientific practice (Davis, 2008).
Preoperative medications
In some cases, the administration of additional drugs before or during anesthesia can significantly enhance the quality of anesthesia and improve the recovery process. The most frequently employed premedications are sedatives and analgesics. Sedatives play a pivotal role in calming the animal, facilitating the smooth induction and recovery phases of anesthesia, and reducing the overall amount of anesthetic agents required. It is worth noting that the use of sedatives before rodent anesthesia is limited due to the added stress it may cause during administration. Moreover, systemic or local analgesics can contribute to reducing the dosage of anesthetic agents needed and preemptively alleviate pain perception, which extends into the recovery period. A valuable resource for guidelines on injectable analgesic agents in rodents is Table 3.
Agent | Species | Dosage (mg/kg)a | Route | Frequency of dosage (hours) |
---|---|---|---|---|
Buprenorphine | Mouse | 0.05-0.1 | IP, SQ | 4-8 |
Rat | 0.05 | IP, SQ | 6-8 | |
Buprenorphine extended-release (ER)b: | Mouse | 0.6/3.25 | SQ | 48/72 |
Zoopharm/Ethiqa XR® c |
Rat | 1.2/0.65 | SQ | 48/72 |
Butorphanold | Mouse | 1-5 | SQ | 2 |
Rat | 2 | SQ | 2 | |
Flunixin (Banamine) | Mouse | 2.5 | SQ | 12-24 |
Rat | 2.5 | SQ | 12 | |
Ketoprofen | Mouse | 10-20 | SQ | 24 |
Rat | 5 | SQ | 24 | |
Carprofen | Mouse | 5/20 | SQ | 12/24 |
Rat | 5 | SQ | 24 | |
Meloxicam/Meloxicam ERe | Mouse | 5-10/6 | SQ | 12/24 |
Rat | 1-2/4 | SQ | 24/72 |
- a
Dosages, sourced from various references, may require individualized adjustments. Typically, analgesics take a few hours to reach their peak plasma concentration. Administering the initial dose before a procedure is often advantageous. The adoption of long-acting analgesics is prevalent in research animal medicine, aiming to prevent or address postoperative or postprocedural pain while minimizing handling-related time and stress. Long-acting formulations suitable for rodents encompass opioids, NSAIDs, and local anesthetics. However, it is important to note that long-acting analgesics may induce skin irritation and injection site reactions, dependent on the specific drug formulation (Huss & Pacharinsak, 2022). Abbreviations: IP, intraperitoneally; SC, subcutaneously.
- b
Buprenorphine/Buprenorphine-ER is a partial agonist that is difficult to reverse if ventilation becomes compromised. It may be prudent to begin with a low published dose and redose if necessary. This is a controlled substance. In rats, this drug increases locomotor activity and may cause pica (indiscriminate appetite, such as eating bedding).
- c
Extended-release buprenorphine (Ethiqa-XR) from Fidelis Animal Health is an FDA-indexed long-acting buprenorphine that is available at a concentration of 1.3 mg/ml
- d
This is a controlled substance with mild analgesic activity in rodents.
- e
Meloxicam-ER is clinically efficacious, but more evaluation is needed for mice and rats (Huss & Pacharinsak, 2022)
Benzodiazepines like diazepam and midazolam, categorized as sedatives, are frequently employed in conjunction with injectable anesthetics to aid in co-induction. While benzodiazepines do not offer analgesic effects, they have minimal impact on the cardiac, pulmonary, renal, and hepatic systems. However, it is important to be aware that benzodiazepines can intensify the respiratory depression caused by opioids. Therefore, careful attention should be given to the choice of injectable anesthetic combinations (Davis, 2008).
α-Adrenoceptor agonists, such as xylazine, medetomidine, and dexmedetomidine, typically provide both sedation and analgesia. Among these combinations, ketamine/xylazine is commonly used for anesthesia in mice and rats. Nevertheless, it is essential to be aware of potential side effects, including bradycardia, hypothermia, and respiratory depression. In cases where additional anesthetic time is required, only the ketamine should be redosed to mitigate the risk of bradycardia and cardiac arrest. It is important to note that in mice and rats, the sedative effects tend to outlast the analgesic effects.
Atropine can be administered approximately 10 min before inducing anesthesia to prevent bradycardia. However, the use of anticholinergics, like atropine, in rodents is a subject of debate, as these interventions are often unnecessary or significantly reduced in rodents. However, if used, it should be noted that while atropine can shield the heart from vagal inhibition or opioid-induced bradycardia, it also has the unintended effect of reducing salivation and increasing the viscosity of airway secretions. In rodents, this increased secretion viscosity may inadvertently obstruct small airways or the trachea. Therefore, when using an anticholinergic, close monitoring of the respiratory pattern is crucial, and if an inhalant is employed, vigorous ventilation of the animal may be necessary. Furthermore, it is important to be aware that the rat rapidly metabolizes atropine due to the presence of hepatic atropine esterase (Davis, 2008).
In summary, the choice of premedication and their administration during anesthesia in rodents should be made carefully, considering the specific needs of the procedure and potential side effects, to ensure the safety and well-being of the animals.
Analgesic drugs
Administering analgesics before surgery reduces the hyperactivity of nerve cells triggered by pain, thereby lowering the requirement for postoperative pain relief medications. Additionally, some analgesics enhance the effects of anesthesia, leading to a significant reduction (often by 40% to 60%) in the amount of inhalant anesthesia necessary for surgical procedures (Flecknell et al., 1990; Hecker et al., 1983).
Opioid analgesia : The strength of opioid binding, or affinity, is linked to the effectiveness of their pain-relieving properties (Basbaum & Levine, 1991). Opioids offer the primary advantage of providing profound pain relief, but they can also lead to CNS depression, which can manifest as lowered body temperature, bradycardia, and respiratory depression. When administered at clinically effective doses, opioids generally have minimal impact on the cardiovascular system of rodents. In cases of severe bradycardia, the use of atropine, typically at a dosage of 0.04-0.1 mg/kg for mice or 0.05 mg/kg for rats, can effectively manage this condition (Flecknell, 1996). There are three major classes of opioid receptors: mu, kappa, and delta. In mice, opioid analgesics targeting the mu-receptor are most often used. Opioid analgesics can further be categorized as either full or partial agonists. The level of pain relief provided by both full and partial mu-agonists is dependent on the dose administered, with partial agonists typically offering less pain relief at their maximum effectiveness compared to full agonists. Butorphanol has mild pain-relieving effects on kappa receptors but acts as a strong antagonist at mu receptors (Flecknell, 1996). Its pain relief lasts for a relatively short duration, typically up to 2 hr (Dyson et al., 2023). In contrast, buprenorphine hydrochloride is a partial mu agonist with a duration of action lasting 4-8 hr. However, the extended-release formula of buprenorphine increases this duration to 48-72 hr. Using buprenorphine can reduce the amount of anesthesia needed, a concept known as ‘anesthetic-sparing,’ and also lowers the required post-operative pain relief. One potential side effect when receiving these analgesics over an extended period is sedation, which may reduce the animal's appetite and water intake. This consideration is important when monitoring the animal's body weight and normal behavior during the post-operative period to assess pain and discomfort. Notably, the extended-release form of buprenorphine can induce pica (a desire to eat non-food substances) in rats (Guillory et al., 2018). As a result, it is advisable to use the extended-release buprenorphine formula in rats for no longer than 48-72 hr post-operatively and closely monitor them to prevent potential ingestion of bedding material (Liles & Flecknell, 1993; Liles et al., 1988).
α2 agonist analgesia : α2 adrenergic agonists (e.g., xylazine, medetomidine, dexmedetomidine) are generally regarded as sedative-hypnotics with a wide range of effects, including sedation, visceral and somatic analgesia, mild to moderate muscle relaxation, peripheral vasoconstriction, bradycardia, and hypothermia. Due to the inhibitory effect on sympathetic outflow, gastrointestinal and endocrine functions are also depressed. Species sensitivity and response to these drugs vary greatly. Xylazine, the most widely used α2 agonist in mice and rats, has a rapid (3-5 min) onset of action and a short duration. Duration of sedation and analgesia is dosage dependent. Mice and rats are sedated for 1-2 hr, but analgesia is brief (15 min). Xylazine should be supplemented with other analgesic agents if prolonged (>30 min) analgesia is needed (Thurmon et al., 1996). Medetomidine, the potent α2 agonist, has fewer side effects because it is more selective for α2 receptors. Although α2 agonist drugs are excellent additions to anesthetic injectable cocktails due to their prolonged sedative effects, their role as sole analgesic agents has yet to be established in mice and rats (Abbott & Bonder, 1997). The detrimental side effects of these drugs can be reversed with the α2 adrenergic antagonist atipamezole (0.1-1.0 mg/kg subcutaneously, intraperitoneally). Also, dexmedetomidine has been identified as the active enantiomer of medetomidine, and the use of dexmedetomidine in combination with ketamine has increased because the dose of dexmedetomidine administered is normally half that of the medetomidine and more predictable sedative effect with lesser cardiovascular side effects (Burnside et al., 2013). However, it is important to carefully consider the advantages and disadvantages of using a reversal agent. Once an α2 adrenergic antagonist is given, the duration of anesthesia is markedly reduced, and recovery of reflexes occurs within 5 min of administration. If given too soon, rapid loss of anesthesia with concomitant loss of muscle relaxation may occur prior to the end of the procedure. Another major disadvantage is the loss of analgesia for animals undergoing a painful procedure. In this situation, the analgesic can be augmented with another opioid or NSAID (see below) before the α2 agonist is reversed. The advantages of using a reversal agent, other than faster recovery time, are a reversal of bradycardia and respiratory depression, thus reducing the occurrence of complications associated with prolonged sedation.
Local anesthesia : Local anesthetics block Na+ channels in cell membranes, preventing depolarization and subsequent activation of peripheral nociceptors, thus blocking pain perception. Properties of the selected anesthetics, size of the dose, and accuracy of injection placement influence the duration of response. To reduce pain from local irritants, such as the ear bars of the stereotaxic apparatus, lidocaine cream (EMLA®) can be placed into the ear canal and on the ear bars. Topical anesthetics can also enhance analgesia during surgery. Infiltrating 0.1-0.2 ml of 0.25% bupivacaine or 1% lidocaine into the skin along the edges of the incision will enhance analgesia and reduce the needed anesthetic dosage. If the use of systemic analgesics is contraindicated by the experimental study, topical anesthesia provides pain relief at the incision site (Durst et al., 2021).
CAUTION : Potential complications (CNS and cardiac toxicity) can result from overdose in smaller rodents after using local anesthetics.
Nonsteroidal anti-inflammatory drugs (NSAIDs) : NSAIDs are a drug class useful for reducing fevers (anti-pyretic), analgesic, platelet inhibitory actions, and anti-inflammatory effects. The anti-inflammatory effects of NSAIDs are achieved by interfering with the arachidonic acid metabolism pathway, specifically targeting the cyclo-oxygenase enzymes COX-1 and COX-2. Inhibition of these COX enzymes disrupt the production of prostanoids and prostaglandins, both of which play a role in inflammatory processes. Typically, NSAIDs undergo metabolic processes in the liver and are excreted through the kidneys. The use of NSAIDs allows faster anesthetic recovery and less post-recovery sedation while providing analgesia. Ketoprofen may be given once daily, beginning with the initial anesthesia. Administering ketoprofen in warm lactated Ringer's solution is an excellent way to provide both fluids and analgesic simultaneously. Fluids provide needed hydration and protect against renal toxicity. Also, NSAIDs are a good choice for craniotomies requiring a stereotaxic apparatus. Generally, post-operative pain is from the mechanical pressure (trauma) of the ear bars, not the craniotomy; NSAIDs are ideal for this type of pain. If NSAIDs (ketoprofen, carprofen, meloxicam) are to be used for a long period of time, it is advisable to include an H2-blocking agent, such as Zantac (0.5 mg/kg IM) or Pepcid (2.5 mg/kg SQ) to mitigate the risk of gastrointestinal damage and provide additional administration of warmed fluids (Wallace et al., 2000).
Oral analgesia _(_Foley et al. , 2019 ) : Concerns about causing additional stress through handling animals following surgery and giving them injections have led many to try oral preparations of analgesic agents. However, voluntary oral consumption of drugs may be impeded by palatability, xenophobia, pain/discomfort, and recovery from anesthesia and generally is ineffective for immediate post-operative use. In addition, the consumption of agents may be influenced by diurnal behavioral rhythms (nocturnal consumption is likely to be greater than that during daylight). Oral doses of these agents may be substantially higher than parenteral doses due to first-pass effects through the liver (i.e., oral doses of buprenorphine and butorphanol are 10 times that of injected doses). Many orally administered agents are only adequate for controlling low-grade pain, and some agents, such as acetaminophen, have little oral potency in rodents. An innovative way of providing oral analgesics to rodents is via flavored gelatin (or water or Nutella). Notably, feeding the gelatin without drugs for a few days conditions the animals to readily accept the drug-impregnated gelatin later. Furthermore, in cases where medication is to be delivered in drinking water, administration for a few days prior to surgery may address the neophobic response. An additional consideration is the method of housing; if more than one animal is housed per cage, one cannot ensure that each animal consumes his or her required dosage of drug. Ultimately, gavage is the only route by which the administration of appropriate doses of oral medications can be ensured (Davis, 2008).
COMMENTARY
Background Information
The size of the mouse or rat presents technically challenging problems that can lead to higher mortality rates due to improper use of anesthesia. Anesthetic complications in rodents can be overcome by becoming familiar with the selected anesthetic agent and its effects on the animal model. This information is especially important when determining dosages of injectable anesthetic agents. Adverse effects of chemical anesthesia are difficult to reverse, while insufficient anesthesia is difficult to safely augment. Published dosage regimens vary widely because many of these protocols come from laboratories with wide differences in the complexity of surgical procedures and skills required. Any published anesthetic dosage regimen must be tailored to the individual animal and experimental procedure.
Critical Parameters
The selection of an anesthetic regimen depends on a variety of factors, as discussed below. Whatever anesthetic regimen is chosen, it is important to remember that the purpose of anesthesia is to prevent pain and provide humane restraint. Selecting the method of anesthesia that is least likely to interfere with the aims of the research is difficult, and the physiological effects of the anesthetic agent should be researched. For example, it may be important to maintain blood pressure within a normal range. Many anesthetic agents maintain blood pressure by stimulating the sympathetic nervous system, so animals have elevated catecholamines. Is this important to the research project? Discussing the method of anesthesia to be used with your veterinary staff prior to submitting your animal protocol is recommended. Finally, although an appropriate anesthetic regimen should be selected for each individual research project, attention must also be given to the management of the anesthetized animal throughout and following the procedure so that the animal does not become hypothermic, hypoxic, or hypercapnic (too much CO2 in blood) (Davis, 2008).
Species variation
Risk is inherent with anesthesia, regardless of the choice of agent or animal species. Wide variation occurs among animal species in their responses to drugs, and extrapolation from one species to another should be avoided. The small size of mice and rats, coupled with the technical difficulties encountered when trying to monitor physiological parameters, increases the risk of anesthesia in these two species. The variability of response to ketamine is particularly relevant. Small dosages of ketamine can immobilize nonhuman primates, but ketamine alone rarely immobilizes ruminants. In rodents, only doses that produce profound respiratory depression are effective, and at these dosages, mortality can exceed 50%. For this reason, ketamine should always be combined with a benzodiazepine or an α2 adrenergic agonist (xylazine) to lower the required ketamine dosage. In addition, dosages must be carefully calculated for each animal because of the small size of rodents, which can vary dramatically between certain stains or genotypes. Dosing based on an average weight will result in uneven anesthesia and possibly death due to the variance of individual animal weights from the average. Most injectable agents must be diluted 5- to 10-fold for accurate dosing (Davis, 2008).
Rodent metabolic rate
The metabolic balance of small animals is precarious. Mice and rats frequently develop metabolic acidosis, hypoglycemia, and electrolyte disturbances when anesthetized. These physiologic responses underscore the vulnerability of small animals and accentuate the detrimental effects of catabolic responses to additional stress triggered by surgery. The high metabolic rate of rodents entails two risk factors. First, higher dosages of an anesthetic agent are required to achieve a stable plane of anesthesia. Second, the drugs are metabolized faster, shortening the duration of anesthesia. If the dose is increased to try and prolong anesthesia, the risk of killing the animal also increases. Aggressive monitoring is critical to avoid complications. It is important to continually monitor the animal's physiologic status, regardless of the anesthetic regimen chosen (Davis, 2008).
Consideration of injectable anesthetic selection and dose
Because health status, age, strain, nutritional status, environment, and route of administration must all be considered, it is difficult to provide precise dosages of recommended injectable agents. A recommended drug dosage that provides adequate depth of anesthesia (loss of reflexes) in one animal is often insufficient in another animal due to animal variability of response to the drug (Ahmed et al., 1989; Flecknell, 1996; Lovell, 1986a,b,c and death from overdosage is often a result of redosing. Some reflexes persist, even when periods of recumbency are long, prompting questions about the adequacy of anesthesia. Published dosages, such as those in Table 2, should be used only as a guide.
To minimize risks, it is recommended that a single animal be anesthetized and the response assessed before larger numbers of animals are used. Dosing should begin at the low end of the suggested dose range, and the animal should be monitored. It may be necessary to try various drug dosages in the context of the experiment and the desired effect. Remember, a significant problem with injectable anesthesia is the inability to adjust the animal's dose once given. Another significant problem with injectable anesthetics is prolonged recovery times. Many physiological factors (e.g., cardiac output) that are often adversely affected by the drug itself influence the pharmacokinetics of injectable anesthesia. For example, several published regimens provide 30-45 min of anesthesia, but sleep time may extend up to 180 min. During this time, the animal is susceptible to hypothermia, respiratory failure, or injury from cage mates if recovering in a group. To avoid this problem, consider an anesthetic regimen that allows the use of a reversal agent to counteract the anesthetic effect (Davis, 2008).
Route of administration (Diehl et al., 2001)
The route of administration of injectable agents largely depends on the formulation of the agent and the manufacturer's recommendations. If multiple choices for the route of administration exist, the choice is largely based on the species and desired duration of effect. The most common route of administration in rodents is intraperitoneal injection. This is generally easy to do and safe if performed correctly. Correct technique is essential to achieve uniform absorption of the anesthetic agent; inadvertent injection into the liver, spleen, or mesenteric fat results in uneven absorption and uneven anesthesia. If properly administered, predictable periods of surgical anesthesia are achieved; however, tissue redistribution of drugs in overweight animals often makes proper anesthesia difficult.
Intramuscular injections in rodents are discouraged. Rodents lack sufficient muscle mass for accurate injection, making the procedure technically demanding. Necrosis and pain are not infrequent outcomes due to the small muscle mass and acidic pH of many agents, such as ketamine. If this route must be used, the location of the sciatic nerve must be determined precisely, and the volume delivered should be limited to ∼0.05 ml/site (mice) or ∼0.1 ml/site (rats) with a smaller needle by dividing the full dosage and performing multiple injections bilaterally in the thigh muscles. The paralumbar muscles provide an additional or alternative site for intramuscular delivery (Gehling et al., 2018).
Subcutaneous injection of anesthesia is attractive due to the ease of administration and the large space between the skin and subcutaneous tissues. The major drawback is uneven, slow absorption, leading to unpredictable levels of anesthesia. For optimal absorption, drugs should be hypo-osmolar (<300 milliosmoles) with a neutral pH. Similar to agents injected intramuscularly, necrosis at the site of entry is a major side effect of highly acidic or basic substances.
Inhalant anesthetics
The most consistent and reliable anesthetic regimens for rodents involve inhalant anesthesia, with two major inhalant anesthetics currently available, namely isoflurane and sevoflurane. Isoflurane and sevoflurane require special equipment such as induction, delivery, and scavenging systems that have impeded their popularity. However, inhalation anesthesia is state-of-the-art, poses fewer risks for the animal, and, for the investigator willing to learn to use the equipment, provides easily controlled anesthesia with remarkably fast induction and recovery times. They also exhibit neuroprotective effects during brain-related conditions like ischemia or infarction and are preferred for rodent models of traumatic brain injury (Dyson et al., 2023). However, their potential for neurotoxicity in specific situations (Neag et al., 2020) means researchers must select them carefully based on their research objectives. Additionally, “Compound A” (fluoromethyl-2,2-difluoro-1-trifluoromethyl vinyl ether), a by-product from the interaction of sevoflurane with soda lime, exhibits nephrotoxicity in rats, though it lacks evidence of neurotoxicity in clinical contexts (Dyson et al., 2023). Sevoflurane's higher cost acts as a deterrent to its usage compared to isoflurane.
Induction chambers and anesthetic delivery
Induction chambers can be purchased or made (Gwynne & Wallace, 1992) from acrylic or other clear materials. Standard induction chambers have a fresh gas inlet and an outlet for exhaled and waste gases. They are clear for easy visualization of the animal. The rodent is placed inside the chamber for induction, then removed from the chamber with anesthesia maintained by delivery through a face mask or nosecone. The chamber should be an appropriate size for the animal (1-2 L size for mouse, or 3-5 L for rat). Smaller chambers allow faster induction times (Davis, 2008).
Both induction chambers and face masks/nosecones require a calibrated vaporizer for precise control of the concentration of anesthetic gas delivered to the animal. Because oxygen is needed to volatilize the liquid anesthetic, oxygen is delivered to the animal and helps maintain blood oxygen saturation. Some vaporizers are designed to deliver one specific agent (e.g., isoflurane) and should not be used to deliver agents with greatly differing vapor pressures (e.g., sevoflurane) since the concentration delivered may vary substantially. In general, a vaporizer setting of 1 × minimal alveolar concentration (1 × MAC) will produce light anesthesia, 1.5 × MAC will produce a surgical depth of anesthesia, and 2 × MAC will produce deep anesthesia. Inhalant anesthesia potency is similar across species, so once an inhalant anesthetic is chosen, the vaporizer settings remain the same (Davis, 2008).
When using a face mask/nosecone, dead space, that part of the circuit that remains filled with expired gas at the end of expiration and is then reinhaled by the animal, should be minimized by using short, straight tubing. The resistance of the anesthetic circuit also influences anesthesia of the animal. The effort that the animal must make to move gas in and out of the circuit can be minimized by avoiding narrow or sharply angled components of the system (Davis, 2008).
An understanding of the animal's physiology will also enable smooth, stable anesthesia. Tidal volume is the volume of gas drawn into the respiratory tract with each breath. The minute ventilation is the volume of gas drawn into the respiratory tract in 1 min, so tidal volume × respiratory rate = minute ventilation. Importantly, the minute ventilation does not equal the gas flow rate needed to keep the animal anesthetized. The rate of fresh gas flow in a system using a face mask should be three times the minute ventilation. If the gas flow rate is too low, the animal will inhale room air from around the edges of the mask, diluting the anesthetic concentration (Davis, 2008).
Waste anesthetic gas (WAG) and gas scavenging
The small size of rodents makes typical veterinary techniques like intubation and ventilation challenging. To address this, induction chambers, face masks/nosecones, use higher gas flow rates, and if performing high-throughput surgeries contributes to waste anesthetic gas (WAG) pollution in lab settings. Even though debate persists about the health effects of occupational WAG exposure and how much focus should be placed on risk reduction, short-term exposure may lead to multiple symptoms (drowsiness, irritability, depression, headache, nausea, and fatigue) stemming from various sources, such as poorly fitted masks/nosecones, leaking connections to the induction box, and poor scavenging system. The most effective means to decrease WAG levels is installing an efficient scavenging system. Active systems, which use a pump to remove anesthetic gases, prove highly effective and are necessary if downdraft tables or fume hoods are not available at the surgery station. Many tabletop options can be commercially purchased. Passive systems, using positive pressure in the anesthetic machine to push WAG into activated charcoal canisters, are common in lab animal settings due to their affordability and portability. However, these canisters need careful monitoring, weighing before and after use, and changing as per manufacturer instructions or when a WAG breakthrough occurs. Ideally, a combination of active and passive scavenging techniques can be employed for enhanced efficiency.
Preoperative evaluation and animal selection
Newly arrived mice/rats should acclimate to their housing room for at least 3 days before any anesthesia procedures. This period allows the stabilization of physiological parameters following shipment. Conducting a thorough preanesthetic animal evaluation is essential to identify underlying issues that might affect anesthesia safety. Preexisting diseases can narrow the therapeutic window of anesthetics, leading to cardiopulmonary depression and other physiological problems. As part of this evaluation, obtaining a detailed history of the colony, including experimental procedures, provides valuable context for assessing the general health status of the animal.
Observing the general behavior of mice/rats in their home cage provides an initial indication of their overall health. Respiratory function can be assessed by observing nares and respiratory pattern, rate, and depth. Abnormalities such as tachypnea, deep abdominal breathing, or gasping indicate poor respiratory capacity and unsuitability for anesthesia. Blood circulation and perfusion can be evaluated by examining mucous membrane color, ear, paw pad, and tail color. Abnormalities such as pale, blue, or bright red mucous membranes may indicate anemia, hypoxia, infection, or circulatory failure.
Dehydration status can be determined by checking for sunken eyes or piloerection, both indicating poor hydration and associated with higher anesthetic mortality. Palpating the sacroiliac bones allows assessment of body condition, providing a more accurate health indicator than weight alone, as certain conditions may increase weight while decreasing muscle and fat. A body condition score (BCS) below 2 suggests poor health, often necessitating euthanasia, while a BCS of 5 indicates an overweight mouse/rat.
Recording the mouse/rat's weight using a properly calibrated scale is essential. This weight measurement is crucial for calculating accurate dosages of anesthetic or analgesic drugs and monitoring rodents during the immediate post-operative and recovery periods. Any abnormal findings from the physical examination indicate compromised health status and render the mouse unsuitable for anesthesia (Navarro et al., 2021).
Perioperative and postoperative monitoring
Anesthesia can disrupt normal balance and may lead to unexpected outcomes, especially in sick individuals or those undergoing complex procedures. These disruptions manifest as alterations in the body's usual physiological functions, forming the foundation of anesthetic monitoring. Monitoring anesthesia enables the identification of any impact on different body systems during a procedure, allowing adjustments to the anesthetic plan to avoid negative effects on the animal in the short and long term. Successful anesthetic monitoring involves three key steps: promptly identifying disruptions to normal balance, accurately interpreting changes, and intervening appropriately (Dyson et al., 2023).
Depth of anesthesia : Monitoring the animal's level of anesthesia is critical to successfully estimating the effectiveness of the dosage and route of administration. The rate and depth of respiration are frequently used to assess cardiopulmonary function. Remember that rodents normally maintain minute ventilation by high respiratory rates and low tidal volumes. During anesthesia, both tidal volume and minute ventilation fall; therefore, the animal should be occasionally ventilated to avoid the risk of respiratory acidosis with or without hypoxemia. Noxious stimuli, such as the toe pinch, will elicit a reflex motor response and quickening of respiration if the level of anesthesia is inadequate.
Rodent surgery is usually done in groups, with several animals operated sequentially. As such, the use of electronic equipment to provide continuous monitoring of physiological variables can be invaluable.
Tissue perfusion and oxygenation : Tissue perfusion and oxygenation status can be effectively evaluated by observing mucous membrane color. It is advisable to assess the overall skin color of their ears, tail, and paw pads.
Changes in skin color can indicate various clinical conditions. Paleness in the skin may signal vasoconstriction, hypotension, hypovolemia, hemorrhage, or hypoxia. Conversely, dark pink skin can result from vasodilation, hypercarbia, or toxic reactions. Severe hypoxemia can manifest as a blue or purple skin color. It is important to note that mice with dark coat colors do not typically have pink ears, tail, or paw pads. However, similar changes in the overall skin hue, such as turning gray, pale, or pink, can still be observed during anesthetic complications. To maintain a sterile surgical field while continuously monitoring skin coloration during surgical procedures, one can use a translucent surgical drape like Press'N Seal Film (Glad Press'N Seal, Glad Products, Oakland, CA) or Tegaderm (3M Corporation, St. Paul, MN). This method ensures effective observation of skin color changes, allowing prompt response to any complications that may arise (Navarro et al., 2021).
Heart rate : Monitoring the heart rate allows early detection of changes associated with cardiovascular system depression or tachycardia during surgical manipulations, indicating an inadequate depth of anesthesia. Noninvasive, portable monitoring modules are commercially available from companies such as Kent Scientific or STARR Life Sciences (Davis, 2008).
Pulse oximetry (SpO2-oxygen saturation) : Pulse oximeters are valuable for monitoring arterial oxygenation and pulse rate. Oxygen saturation should always exceed 95% (SpO2). Detection of arterial hypoxemia provides a “common pathway” to detect problems of different origin (hypoventilation, airway obstruction, equipment-related problems). However, pulse oximeters provide no information about CO2 concentration and, therefore, are of little use in warning of respiratory failure due to CO2 retention. Several pulse oximeters are available for use in small animals, although the low volume of tissue available for monitoring limits their use in small animals (Alexander et al., 1989; Vender et al., 1995).
Respiratory pattern : Before administering anesthesia, it is essential to assess normal respiration to establish a baseline. This allows for the evaluation of any changes in respiratory rate (RR), pattern, and depth after the induction of anesthesia. RR can be assessed by counting the rise and fall of the chest over 1 min, providing the breaths per minute measurement. It is crucial to keep in mind that both an excessively high or low RR can signal significant issues. An RR that is too low or characterized by very shallow breathing may indicate that the anesthetic plane is excessively deep. Conversely, an elevated RR could suggest that the animal is too light, potentially experiencing pain, or not adequately anesthetized (Navarro et al., 2021).
pH : Disturbances in systemic pH are common and can be minimized by shortening anesthesia time.
Capnography : Capnography measures the concentration of exhaled CO2 during the respiratory cycle in intubated or ventilated rodents. It provides real-time End-tidal CO2 (ETCO2) data and serves as an important tool for alerting anesthetists to apnea events during the ventilation process. There are three main types of capnography equipment available, including those offered by Kent Scientific (CapnoScan), Harvard Apparatus (type 430), and ITIC Life Sciences. (MicroCapStar). High ETCO2 levels, known as hypercapnia, can indicate hypoventilation, which might be caused by factors like a deep anesthetic plane, respiratory depression from opioid use, patient positioning, or obesity. Conversely, low ETCO2 levels, termed hypocapnia, suggest hyperventilation, which may result from reduced cardiac output, lowered blood pressure, decreased pulmonary perfusion, or the presence of a thromboembolism. In mice, due to their rapid respiration and small tidal volume, interpreting capnograph waveforms can be challenging. However, monitoring ETCO2 levels is invaluable for alerting anesthetists to potential issues with equipment or the patient's condition (Navarro et al., 2021).
Blood pressure : Blood pressure refers to the force exerted by circulating blood on the walls of blood vessels, allowing us to estimate cardiac output and evaluate tissue perfusion. The measurement of blood pressure can be achieved using either direct or indirect methods. Direct measurement involves the surgical insertion of a catheter into an exposed artery. In contrast, indirect measurements utilize inflatable tail pressure cuffs designed for rodents, such as those offered by Kent Scientific or Harvard Apparatus. While indirect monitoring is more straightforward to perform, it provides only intermittent data on pressure fluctuations. In small rodents, the accuracy of blood pressure measurements is compromised due to their high pulse rate and reduced arterial pulse. Furthermore, maintaining a warm environment for mice/rats is crucial during tail-cuff plethysmography to ensure sufficient blood flow to the tail and accurate measurements (Navarro et al., 2021).
Perioperative and postoperative supportive care
Rodents are subject to hypothermia due to their high metabolic rate and high body surface area to body weight ratio. This vulnerability is heightened by the removal of hair and dampening of the remaining fur during the aseptic preparation for surgery. Placing this small, wet animal on a cold metal surface can lead to potentially fatal hypothermia. Opening the body cavity during surgery also hastens the loss of body heat (Dyson et al., 2023). In addition, most anesthetic agents depress the thermoregulatory centers of the brain. The longer the animal is anesthetized, the greater the risk of lowering core body temperature. Hypothermia, the most common cause of delayed recovery from anesthesia, delays anesthetic metabolism and urinary excretion of the anesthetic agent. The metabolic rate falls, and the residual injectable anesthesia effectively reanesthetizes the animal. Unfortunately, mice and rats lack the physiological ability to overcome these events, and the outcome is frequently death. Recirculating warm water recirculating blankets, feedback-controlled or infrared warming pad, forced warm air units, warm fluid SQ/IP lavage intraoperatively, pocket warmers, and plastic wrap for insulation all provide heat sources necessary for safe maintenance and recovery of the animal. Recirculating warm (35-36°C) water blankets are safer than electrical heating pads because there is no risk of burning the animal. Utilizing a rectal probe equipped with a thermistor thermometer proves effective in monitoring the core body temperature of rodents. Crucially, maintaining continuous monitoring throughout both anesthesia and recovery, with regular assessments of anesthetic depth and respiratory rate at intervals of every 15 min, is imperative. A common, often fatal, mistake (due to hypothermia) is mistaking sternal recumbency for full recovery and prematurely returning the animal to the animal holding room, which is probably cooler than the surgical room. If individually ventilated cages (IVCs) are used, the forced ventilation at the cage level further cools the animal. To facilitate speed of recovery, the rodent's cage can be placed on warming devices, a slide warmer, or a recirculating warm water blanket. However, it is important to warm no more than one-half of the recovery cage, allowing animals to move freely to the cooler side when they regain ambulation to prevent overheating. Care should be taken to extend the neck to help with breathing, and if the animal is placed on bedding, a piece of gauze or cloth should be placed under the animal's mouth to prevent accidental inhalation of bedding. Moreover, animals should be kept separated from other recovered (or awake) animals to prevent possible injury until each animal is fully awake and ambulatory.
Surgical procedures, even if minor, result in fluid loss due to high metabolic rate, exposure of mucous membranes, open body cavities, and possibly hemorrhage. Fluid therapy should be considered for every surgical procedure. Warmed fluids can be given subcutaneously and can also contain an analgesic that will aid in postoperative pain management. Investigators should consult with their veterinarian to determine the dosage and frequency of fluid administration.
In the postoperative period, monitoring the surgical site is crucial. Cage-side observation is necessary to detect signs of dehiscence and infection, which can arise from issues like surgical technique, inadequate aseptic practices, or rodents gnawing or licking the incision site. Proper analgesic protocols play a significant role in minimizing the risk associated with behavioral issues.
Postoperative nutrition : Mice and rats have high energy needs. Nutritional support is critical for recovery to avoid hypoglycemia. Volume deficits can be corrected by subcutaneous or intraperitoneal injection of warmed lactated Ringer's solution or 0.9% NaCl, and animals recovering from surgery benefit from additional nutritional supplements such as liquid enteral nutrition, dietary gel or bacon softies (Davis, 2008).
Evaluating analgesic efficacy
Normal physiological behaviors, such as activity, eating and drinking patterns, and weight gain, are parameters frequently used to determine if an animal is experiencing pain and if the chosen analgesic is efficacious. Alternatively, more advanced methods, such as recording and evaluating mouse/rat grimace scores, nest complexity, the Time to Incorporate to Nest Test (TINT), and burrowing behavior, have been developed as rapid tools for pain assessment. It is important that investigators become familiar with the normal behavior of their animal model while realizing that easily recognized signs of pain will rarely be present. Two problems impede species familiarity: (1) rodents are nocturnal (active at night), so observations made during the day may be inaccurate; and (2) rodents, unlike domesticated species, do not respond positively to human contact, often remaining immobile during observation (Davis, 2008). Moreover, every pain assessment method has its strengths and weaknesses. To best assess pain in rodents, it is most effective to combine different methods, including looking at their physical data and behaviors. Considering these indicators can help alert caretakers when animals need medical attention and signal that they might require more pain relief and monitoring.
Body weight and food/water intake are frequently depressed when rodents are distressed or in pain, so these are popular parameters to monitor for evidence of pain. Decreased food/water intake triggers a cascade of events, including dehydration (reduced water intake), hypoglycemia (sluggishness), and hypothermia (insufficient caloric intake and minimal locomotor activity). Returning to normal food and water consumption upon administration of an analgesic is a good sign; however, behavioral changes may occur following analgesia alone (Davis, 2008).
Analgesics, like anesthetics, also evoke a wide variety of responses. It is important that the researcher recognize that some parameters should not be used as evidence of analgesic efficacy with certain analgesics. Buprenorphine influences normal locomotor behavior in rats (Liles & Flecknell, 1993); therefore, this parameter should not be used as evidence of buprenorphine's efficacy. In contrast, NSAIDs do not influence locomotor activity in rats; therefore, this parameter could be used to assess the efficacy of an NSAID in pain management.
Finally, in addition to considering the appropriate selection and use of both anesthetics and analgesics for an experimental procedure, it is important to recognize that the degree of pain and distress experienced by the animal will be markedly influenced by the expertise of the person performing the procedure(s), including simple handling and restraint. This highlights the ethical obligation of the scientist to ensure that all involved in the research project have received adequate training and have demonstrated competence in the procedures required (Davis, 2008).
Troubleshooting
Table 4 lists some problems that may be encountered with the use of anesthetics and analgesics in rodents. Also provided are explanations of the causes of the problems and suggested solutions for avoiding or overcoming them. The most common difficulties occur from overdosage of anesthesia.
Problem | Possible cause | Solution |
---|---|---|
Bradycardia |
Deep anesthetic plane High vagal tone Drug overdose (i.e., opioids, α-2 agonists) |
Lighten anesthetic plane (or stop isoflurane) Atropine drop on the tongue Inject α₂ adrenergic receptor antagonist (atipamezole) Clear airway (suction mouth cavity and pull-out tongue) Provide 100% oxygen In the case of cardiovascular failure, give 0.1 mg epinephrine and gentle, rapid compression of the thorax between thumb and forefinger |
Hypoventilation, respiratory arrest |
CNS depression due to anesthetic depth and drugs Limited thoracic wall Traumatic injuries or upper airway obstruction Recent hyperventilation (too low CO2) |
Clear airway Adjust anesthetic plane Place animal in palm of hand and tip its head down and up. Movement of abdominal contents against the diaphragm will compress and expand the chest. Place a drop of doxapram (respiratory stimulant) on the tongue when the animal takes a breath |
Hyperventilation | lightening level of anesthesia |
Check the vaporizer, oxygen tank, and anesthesia circuits if using inhalant anesthesia. Redose anesthetic agent if using injectable anesthetics |
Hypoxia (blue membranes), <85% oxygen saturation |
Hypoventilation Empty oxygen tank Anesthetic and breathing circuit failure (obstruction, rebreathing expired gas) |
Clear airway Provide 100% oxygen Stop vaporizer Correct inhalant anesthetic circuit Consider administering reversal agents (atipamezole for xylazine or naloxone for opioids) if using injectable anesthetic agents |
Hypothermia |
Decreased thermoregulation by anesthetic agents Non-rebreathing circuits with high O2 flow Large surgical area with longer procedures No heat support |
Provide heat support Minimize area of removed hair Reduce surgical and anesthetic time Administer warm fluid |
Cardiopulmonary failure |
Bradycardia, hypotension, hypoventilation, apnea, and hypothermia Surgical manipulation |
Clear airway CPR (chest compression - hold the chest between thumb and finger, rapidly compress the area over the heart ∼90 times/min) Consider 0.1 mg epinephrine Provide 100% oxygen Consider reversal agents once respiration and heartbeat have returned |
Acknowledgments
This work builds upon Protocols in Rodent Anesthesia and Analgesia, published by Dr. Judith A. Davis in 2008; we are indebted to Dr. Davis for laying the foundation of this important material.
The authors are supported by the NINDS/NIH IRP.
Author Contributions
Sang Su Oh : Writing—original draft. Heather L. Narver : Writing—review and editing.
Conflict of Interest
The authors declare they have no conflict of interest.
Open Research
Data Availability Statement
Data sharing not applicable – no new data generated, or the article describes entirely theoretical research.
Literature Cited
- Abbott, F. V., & Bonder, M. (1997). Options for management of acute pain in the rat. The Veterinary Record , 140, 553–557. https://doi.org/10.1136/vr.140.21.553
- Adelsperger, A. R., Bigiarelli-Nogas, K. J., Toore, I., & Goergen, C. J. (2016). Use of a low-flow digital anesthesia system for mice and rats. Journal of Visualized Experiments: JoVE , (115), 54436.
- Ahmed, F., Lundin, G. G., & Shire, J. G. M. (1989). Lysosomal mutations increase susceptibility to anesthetics. Experientia , 45, 1133–1135. https://doi.org/10.1007/BF01950180
- Alexander, C. M., Teller, L. E., & Gross, J. B. (1989). Principles of pulse oximetry: Theoretical and practical considerations. Anesthesia and Analgesia , 68, 368–376. https://doi.org/10.1213/00000539-198903000-00035
- Anderson, L. C., Fox, J. G., Otto, G., Pritchett-Corning, K. R., & Whary, M. T. (2015). Laboratory animal medicine ( 3rd ed.). Academic Press.
- Basbaum, A. I., & Levine, J. D. (1991). Opiate analgesia. New England Journal of Medicine , 325, 1168–1169. https://doi.org/10.1056/NEJM199110173251610
- Bennett, K., & Lewis, K. (2022). Sedation and anesthesia in rodents. The Veterinary Clinics of North America. Exotic Animal Practice , 25(1), 211–255. https://doi.org/10.1016/j.cvex.2021.08.013
- Burnside, W. M., Flecknell, P. A., Cameron, A. I., & Thomas, A. A. (2013). A comparison of medetomidine and its active enantiomer dexmedetomidine when administered with ketamine in mice. BMC Veterinary Research , 9, 48. https://doi.org/10.1186/1746-6148-9-48
- Davis, J. A. (2008). Mouse and rat anesthesia and analgesia. In Current Protocol in Neuroscience. Wiley.
- Diehl, K. H., Hull, R., Morton, D., Pfister, R., Rabemampianina, Y., Smith, D., Vidal, J. M., & van de Vorstenbosch, C. (2001). A good practice guide to the administration of substances and removal of blood, including routes and volumes. Journal of Applied Toxicology , 21(1), 15–23. https://doi.org/10.1002/jat.727
- Donovan, J., & Brown, P. (2005). Parenteral injections. Current Protocols in Neuroscience , 33, A.4F.1–A.4F.9. https://doi.org/10.1002/0471142301.nsa04fs33
- Durst, M. S., Arras, M., Palme, R., Talbot, S. R., & Jirkof, P. (2021). Lidocaine and bupivacaine as part of multimodal pain management in a C57BL/6J laparotomy mouse model. Scientific Reports , 11(1), 10918. https://doi.org/10.1038/s41598-021-90331-2
- Dyson, M., Jirkof, P., Lofgren, J., Nunamaker, E., & Pang, D. (2023). American College of Laboratory Animal Medicine Series ( 3rd ed.). Academic Press.
- Flecknell, P. A. (1996). Laboratory animal anesthesia ( 2nd ed.). Academic Press.
- Flecknell, P. A., Kirk, A. J. B., Fox, C. E., & Dark, J. H. (1990). Long-term anesthesia with propofol and alfentanil in the dog and its partial reversal with nalbuphine. Journal of the Association of Veterinary Anaesthetists of Great Britain and Ireland , 17, 11–16. https://doi.org/10.1111/j.1467-2995.1990.tb00381.x
- Foley, P. L., Kendall, L. V., & Turner, P. V. (2019). Clinical management of pain in rodents. Comparative Medicine , 69(6), 468–489. https://doi.org/10.30802/AALAS-CM-19-000048
- Gehling, A. M., Kuszpit, K., Bailey, E. J., Allen-Worthington, K. H., Fetterer, D. P., Rico, P. J., Bocan, T. M., & Hofer, C. C. (2018). Evaluation of volume of intramuscular injection into the caudal thigh muscles of female and male BALB/c Mice (Mus musculus). Journal of the American Association for Laboratory Animal Science: JAALAS , 57(1), 35–43.
- Gwynne, B. J., & Wallace, J. (1992). A modified anesthetic induction chamber for rats. Laboratory Animals , 26, 163–166. https://doi.org/10.1258/002367792780740620
- Guillory, A. N., Clayton, R. P., Prasai, A., Jay, J. W., Wetzel, M., El Ayadi, A., Herndon, D. N., & Finnerty, C. C. (2018). Buprenorphine-sustained release alters hemodynamic parameters in a rat burn model. Journal of Surgical Research , 232, 154–159. https://doi.org/10.1016/j.jss.2018.03.016
- Hecker, B. R., Lake, C. L., DiFazio, C. A., Moscicki, J. C., & Engle, J. S. (1983). The decrease in minimum alveolar concentration produced by sufentanil in rats. Anesthesia and Analgesia , 62, 987–990. https://doi.org/10.1213/00000539-198311000-00005
- Huss, M. K., & Pacharinsak, C. (2022). A review of long-acting parenteral analgesics for mice and rats. Journal of the American Association for Laboratory Animal Science: JAALAS , 61(6), 595–602. https://doi.org/10.30802/AALAS-JAALAS-22-000061
- Kufoy, E. A., Vytautas, A. P., Parks, C. D., Wells, A., Yang, C., & Fox, A. (1989). Keratoconjunctivitis sicca with associated secondary uveitis elicited in rats after systemic xylazine/ketamine anesthesia. Experimental Eye Research , 49, 861–871. https://doi.org/10.1016/S0014-4835(89)80045-4
- Liles, J. H., & Flecknell, P. A. (1993). The effects of surgical stimulus on the rat and the influence of analgesic treatment. British Veterinary Journal , 149, 515–525. https://doi.org/10.1016/S0007-1935(05)80036-3
- Liles, J. H., Flecknell, P. A., Roughan, J., & Cruz- Madorran, I. (1988). Influence of oral buprenorphine, oral naltrexone or morphine on the effects of laparotomy in the rat. Laboratory Animals , 32, 149–161.
- Lovell, D. P. (1986a). Variation in pentobarbitone sleep time in mice. 1. Strain and sex differences. Laboratory Animals , 20, 85–90. https://doi.org/10.1258/002367786780865142
- Lovell, D. P. (1986b). Variation in pentobarbitone sleep time in mice. 2. Variables affecting test results. Laboratory Animals , 20, 91–96. https://doi.org/10.1258/002367786780865089
- Lovell, D. P. (1986c). Variation in barbiturate sleeping time in mice. 3. Strain X environment interactions. Laboratory Animals , 20, 307–312. https://doi.org/10.1258/002367786780808802
- Mitzner, W., Brown, R., & Lee, W. (2001). In vivo measurement of lung volumes in mice, Physiol. Genomics , 4(3), 215–221.
- Nair, A. B., & Jacob, S. (2016). A simple practice guide for dose conversion between animals and human. Journal of Basic and Clinical Pharmacy , 7(2), 27–31. https://doi.org/10.4103/0976-0105.177703
- Navarro, K. L., Huss, M., Smith, J. C., Sharp, P., Marx, J. O., & Pacharinsak, C. (2021). Mouse anesthesia: The art and science. ILAR Journal , 62(1-2), 238–273. https://doi.org/10.1093/ilar/ilab016
- Neag, M. A., Mitre, A. O., Catinean, A., & Mitre, C. I. (2020). An overview on the mechanisms of neuroprotection and neurotoxicity of isoflurane and sevoflurane in experimental studies. Brain Research Bulletin , 165, 281–289. https://doi.org/10.1016/j.brainresbull.2020.10.011
- Smith, W. (1993). Responses of laboratory animals to some injectable anesthetics. Laboratory Animals , 27, 30–39. https://doi.org/10.1258/002367793781082377
- Thurmon, J. C., Tranquilli, W. J., & Benson, G. J. (1996). Preanesthetics and anesthetic adjuncts. In J. C. Thurmon, W. J. Tranquilli, & G. J. Benson (Eds.), Lumb and Jones’ veterinary anesthesia ( 3rd ed., pp. 183–209). Williams & Wilkins.
- Turner, P. V., & Albassam, M. A. (2005). Susceptibility of rats to corneal lesions after injectable anesthesia. Comparative Medicine , 55, 175–182.
- Vender, J. R., Hand, C. M., Sedor, D., Tabor, S. L., & Black, P. (1995). Oxygen saturation monitoring in experimental surgery: A comparison of pulse oximetry and arterial blood gas measurement. Laboratory Animal Science , 45, 211–215.
- Wallace, J. L., McKnight, W., Reuter, B. K., & Vergnolle, N. (2000). NSAID-induced gastric damage in rats: Requirement for inhibition of both cyclooxygenase 1 and 2. Gastroenterology , 119(3), 706–714. https://doi.org/10.1053/gast.2000.16510
Internet Resources
This is an excellent site for finding information about rodent surgery-related equipment and other resources (training and publication).
This is an excellent site for all product information related to rodent surgery, including active scavenging systems and rodent intubation products.
This is an excellent site for finding information about rodent surgical and stereotaxic instruments.
This is another excellent site for all information about rodent surgical and stereotaxic instruments.
This is an excellent site for finding information about rodent surgery-related equipment, such as multiple animal surgery circuits, active scavenging systems, and ventilators.
Citing Literature
Number of times cited according to CrossRef: 2
- Marina Stavrou, Elena Georgiou, Kleopas A. Kleopa, Lumbar Intrathecal Injection in Adult and Neonatal Mice, Current Protocols, 10.1002/cpz1.1091, 4 , 6, (2024).
- Shuangshuang Liu, FangYue Liu, Zhaoxiaonan Lin, Wei Yin, Sanhua Fang, Ying Piao, Li Liu, Yi Shen, Identification of cortical arteries and veins in awake mice using two‐photon microscopy, Journal of Anatomy, 10.1111/joa.14110.